Blue-Green Infrastructure for All Seasons: The Need for Multicolored Thinking
Publication: Journal of Sustainable Water in the Built Environment
Volume 8, Issue 4
Abstract
Forum papers are thought-provoking opinion pieces or essays founded in fact, sometimes containing speculation, on a civil engineering topic of general interest and relevance to the readership of the journal. The views expressed in this Forum article do not necessarily reflect the views of ASCE or the Editorial Board of the journal.
Introduction
With space in urban environments often at a spatial and economic premium, increasing attention is given to the inclusion of infrastructure, which offers multiple benefits to urban residents. Kick-started by the United Nations (UN) Millennium Ecosystem Assessment (UN MEA 2005), the use of an ecosystem service (ES) approach has raised awareness of the multiple services and benefits delivered by the environment and their role in sustaining human health and well-being. Increasing numbers of studies have focused on identifying, quantifying, and economically valuing the delivery of a range of ESs (UK NEA 2011). Implementation of the approach is also promoted as a way to contribute to achieving international (e.g., UN Sustainable Development Goals), European (e.g., the European Green Deal’s zero pollution ambition), and national (sustainable planning) requirements. Within urban areas, several studies have evaluated the delivery of a range of ESs by urban blue-green infrastructure (BGI) (also referred to as nature-based solutions) whose primary function is the mitigation of surface runoff quantity and quality (e.g., Ashley et al. 2018). As a concept, BGI goes beyond stormwater (Fletcher et al. 2015), with authors such as Wright (2011) highlighting that connectivity, natural elements, and multifunctionality are core concepts of (blue-)green infrastructure. Consequently, BGI has been promoted as an opportunity to contribute to healthier urban lifestyles, create economic value, increase the resilience of urban spaces in the face of a rapidly changing climate, and reduce the impacts of polluted stormwater on receiving waters (Tzoulas et al. 2007; Fletcher et al. 2015; Ashley et al. 2018).
Though a range of types of BGI have been implemented within a diversity of environments and climates, to date its conceptualization has been a “one size fits all” in that ESs and benefits derived from the blue and green components have been the focus of research and practice. However, in many regions, blue-green spaces are neither blue nor green for extended time periods but, e.g., white (snow covered) or yellow/brown (dormant vegetation or drought). The implications of this for BGI systems at locations outside the temperate climate zones are yet to be robustly evaluated regarding both their technical-environmental functioning and the delivery of ESs.
Hence, in this paper we propose a novel theoretical framework to expand the BGI concept for consideration of ESs from BGI during nonblue or nongreen seasons. To illustrate this conceptualization, the focus of this paper is white spaces, with the aim of supporting an evaluation of the year-round performance of blue-green-white infrastructure (BGWI). The role of white space in the performance of BGI has yet to be addressed in any context. As a contribution to addressing this knowledge gap, this paper evaluates the delivery of ESs by BGWI in general and identifies the challenges and opportunities provided by the extension of the BGI concept to BGWI. The theoretical discussion is based on previous literature regarding BGI in relation to snow and ES, and a “real-world” BGWI example is illustrated in a Swedish context through reference to a Blue-Green-White Structural Plan (Gällivare Municipality 2016) developed for Gällivare (northern Sweden). However, the perspectives in this paper should not be limited to Sweden but rather expanded to include all possible urban environments, particularly in locations with demanding climates.
Blue-Green-White Infrastructure and ESs
The term BGWI is applicable to parts of North America, Scandinavia, Iceland, and Russia, as well as mountainous regions that have reoccurring periods of snow cover. In such regions, the cold climate with long, snow-rich winters sets different preconditions and requirements for BGI. Consequently, one of the main challenges that cities in such regions need to manage is sustaining the functionality of urban infrastructures and ES providers across seasons due to dramatic changes in temperature and the quality of precipitation from summer to winter.
The types and relative contributions of green, blue, and white spaces over an annual time period are identified in Fig. 1(a) using a Northern Scandinavian climate as an example. During winter, stormwater BGI is snow covered and, hence, provides white—as opposed to blue-green—space. As illustrated in Fig. 1(a), the blue-green season comprises only a minor part of the year. Irrespective of this, the term BGI is commonly used in integrated stormwater management in subarctic regions, effectively neglecting both challenges and opportunities of the “white” season and space. For example, the annual range in temperatures (by up to as much as 60°C) affects the need for and type of stormwater management required whereby rainfall is replaced by snowfall and snow becomes stormwater during the melt season when large amounts of water are released. Such snowmelt requires management in April, just as the green structure has just started to recover after winter dormancy.
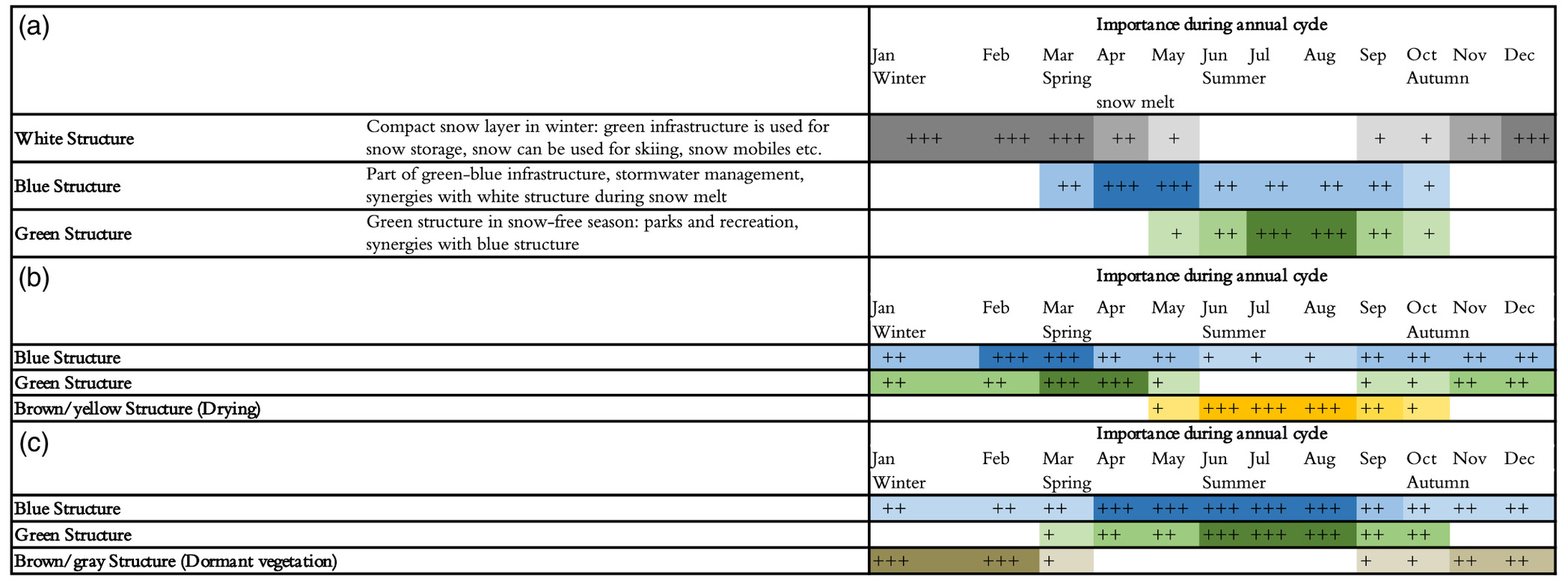
Identified as an abiotic component of ecosystem, only limited information is available on the delivery of ESs by abiotic components (van der Meulen et al. 2016), with even less consideration of the ES delivered specifically by snow (Smith et al. 2017). In Table 1, an overview of the role of potential ESs provided by BGWI in comparison to those provided by BGI is presented.
ES categories | Types of ESs | BGI | BGWI |
---|---|---|---|
Supporting services | Primary production | C locked up by vegetation () | Snow algal communities importance as primary producers (Davey et al. 2019) |
Production of oxygen | Oxygen produced by vegetation () | Snow algal communities importance as oxygen producers | |
Soil formation | Soil generated () | — | |
Water cycling | Contribution to water cycle functioning (percentage permeability of area) | Contribution to water cycle functioning (percentage permeability of area) | |
Provisioning of habitat | Habitat provided (ha) | Habitat provided for arctic and non-Arctic species (ha) | |
Provisioning services | Food | Fish, fruit, and vegetables | — |
Water | Potable and nonpotable water | Potable and nonpotable water (Smith et al. 2017) | |
Renewable energy | Hydropower | Indirectly via meltwaters; storing snow for summer cooling (Nordell 2015) | |
Genetic resources | Pollutant degrading microbial consortia | Cyrospheric pollutant degrading microbial consortia (Mortazavi et al. 2019) | |
Regulating services | Climate regulation | Reduced urban temperatures | Influences levels of albedo (Lutz and Howarth 2015) |
Water regulation | Reduced runoff volume/velocity | Seasonal water storage | |
Erosion control | Stabilization of sediments | — | |
Water purification | Removal of pollutants | Traps pollutants; slow release of melt water at low flow velocities traps coarser particles | |
Cultural services | Spiritual value | Mental health well-being | Mental health well-being |
Educational value | Increased environmental awareness | Safe space to be outside | |
Aesthetics | Sense of tranquility | Sense of tranquility | |
Recreation | Physical well-being | Ski tracks, snowmobile tracks, sledging |
The year-round ES delivery under dramatic changes of temperatures and the nature of precipitation events adds one more dimension (winter–summer component), or color, to the challenge of integrated planning, i.e., stormwater and snow management. However, the white dimension can also be considered an ES asset [Fig. 1(a)]. Hence, the possibility of introducing the integrated blue-green-white dimensions in subarctic urban areas is justified as part of a holistic assessment of system functionality.
Though green structures are used for stormwater management in summer, these areas are less relevant for water management in winter since any precipitation falls as snow. During that season, the structures provide local snow deposit areas. Green corridors serve for bike and pedestrian transportation both during summer and winter (Fig. 2). Fig. 2 shows a model street section with the integration of BGWI and possible functions and services in both winter and summer. Though it is a common, partly multifunctional BGI system in summer, when it comes to winter mobility, local snow storage can be combined with winter paths for, e.g., snowmobiles and as meeting places such as playgrounds. For this, surfaces not needed for water management in winter (as no water flows) can be used. It is suggested that BGWI where snow is stored is seen as an opportunity instead of solely targeting storage, as illustrated in Fig. 3, where the snow stored on the swale is prepared as a ski track.
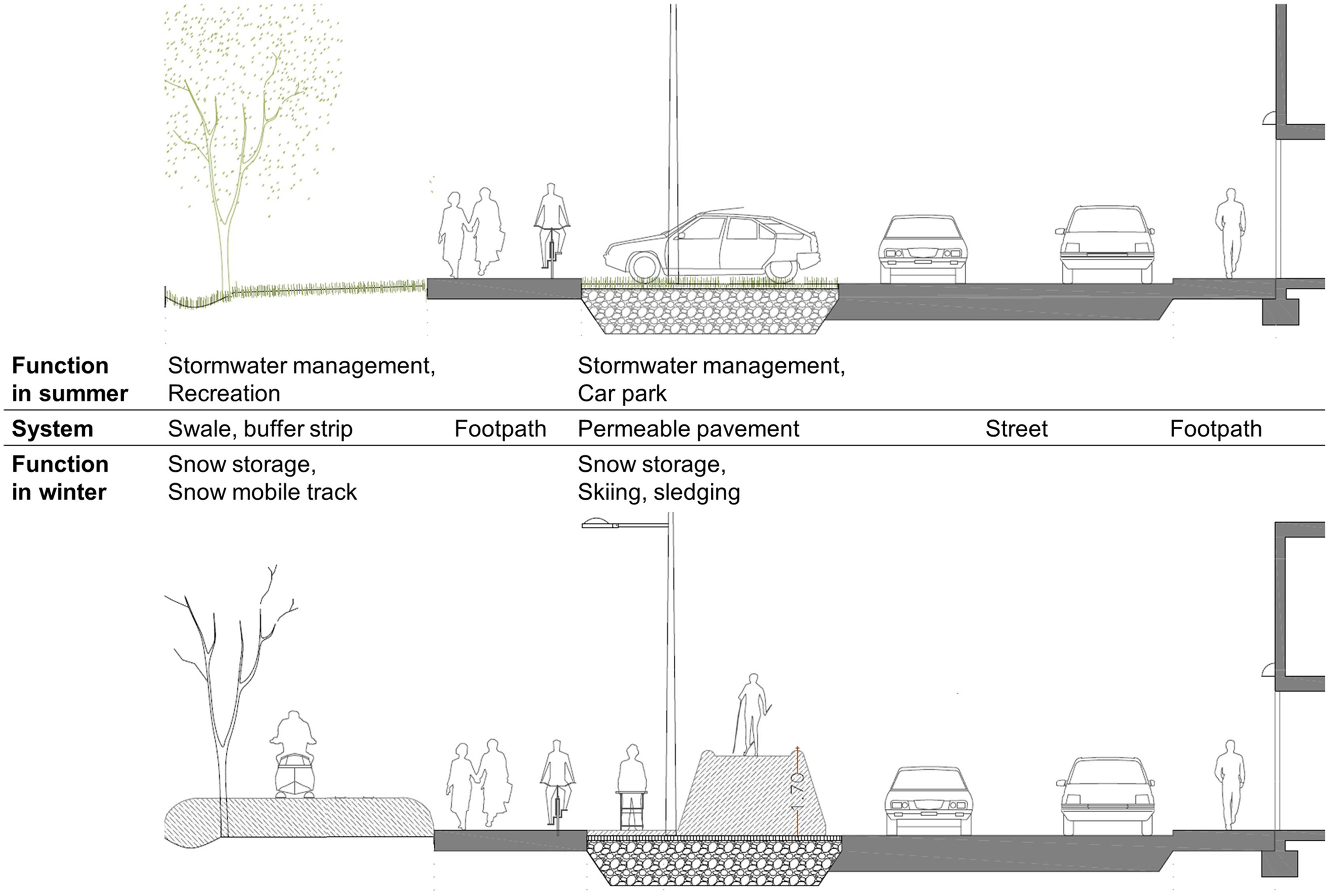
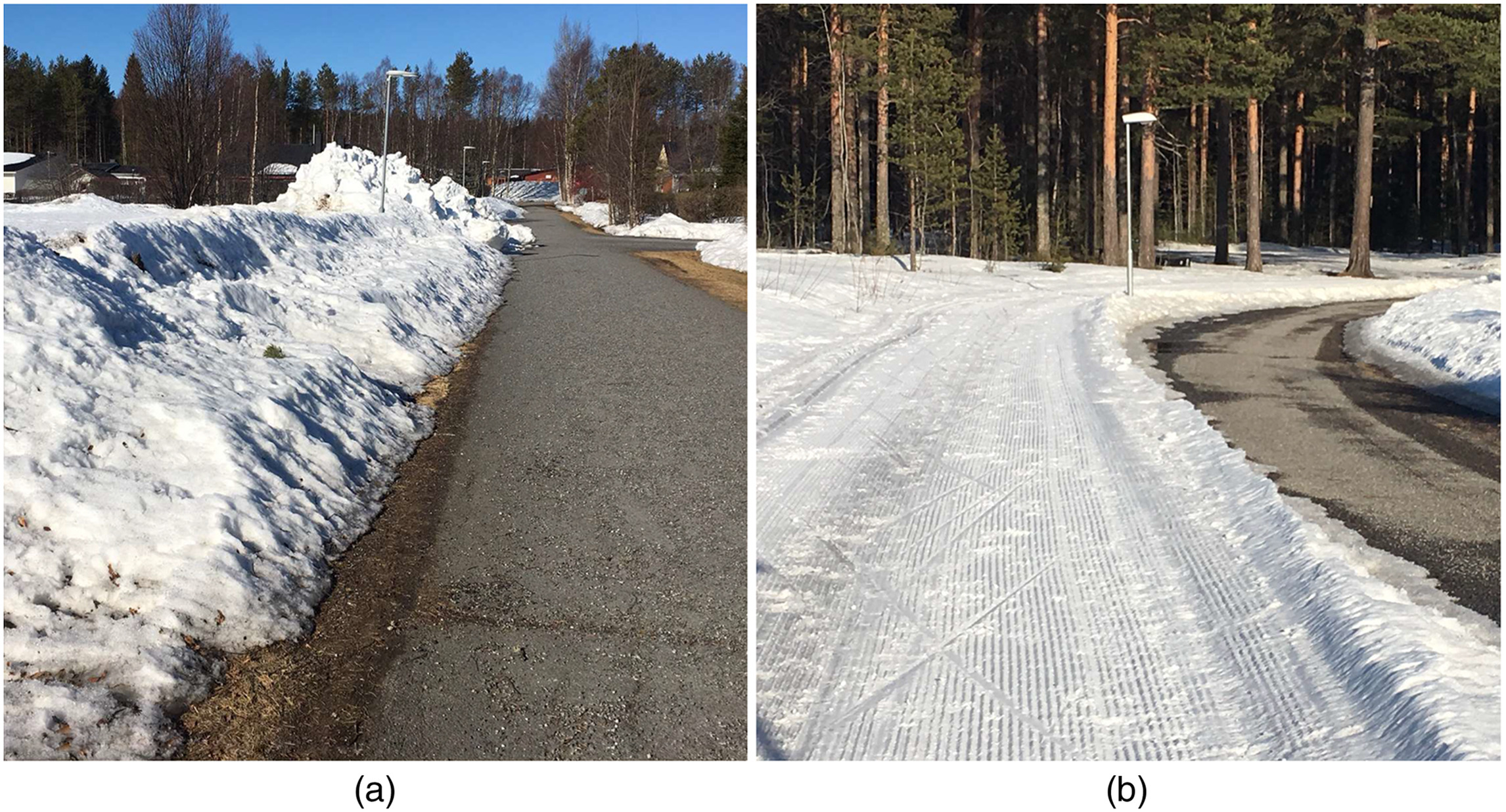
Technical Functionality of BGWI
Concerns over the technical functionality of BGI in cold climates have been raised, and a range of impacts have been identified, e.g., ice covers in ponds and wetlands leading to oxygen depletion and changed flow patterns (German et al. 2003), less efficient (bio)chemical processes in bioretention cells (Muthanna et al. 2007), the limited number of adapted plant species (Lönnqvist et al. 2021), road salt affecting BGI functionality (Søberg et al. 2014), less evapotranspiration during cold seasons (Johannessen et al. 2017), and reduced infiltration due to clogging caused by abrasives used for winter road maintenance or frozen facilities (Moghadas et al. 2016). However, studies have also shown that despite these impacts, a range of BGI (e.g., ponds, bioretention cells, and green roofs) continue to have a positive impact on stormwater quality and quantity objectives (e.g., Marsalek et al. 2003; Muthanna et al. 2007; Johannessen et al. 2017; Kratky et al. 2017; Søberg et al. 2014; Tahvonen 2018; Kuoppamäki 2021). As a contribution to improved urban planning in subarctic regions, the BGWI concept is an asset that has the potential to resolve technical issues related to BGI in cold climates and at the same time present an opportunity to derive ESs and benefits as a contribution to health and well-being objectives (Table 1).
Challenges
The subarctic climate poses specific challenges for all urban infrastructures, including (blue-green) stormwater infrastructure. For instance, seasonal snow cover requires snow removal from parts of public spaces, and the removed snow must be stored somewhere until it melts. Snow management is thus also stormwater management in subarctic regions (Vijayan et al. 2019). Furthermore, given that urban snow accumulates pollutants (and is hence sometimes black or gray rather than white), this issue must be regarded in such a context. Over 15 years ago, Reinosdotter and Viklander (2006) proposed snow separation strategies that distinguished between heavily polluted and less polluted snow to reduce environmental impacts associated with snowmelt. When using snow to deliver ES, implementing such strategies is even more important.
Additionally, already when implementing multifunctional BGI, regulations and unclear/competing responsibilities are challenging (Ellis and Lundy 2016). Often, the roads department is responsible for snow management, which is, thus, often disconnected from water management, and this is challenging because of the high level of codependence between white, blue, and green infrastructures, underlining the need for institutional reform to facilitate the integration of different functions (Brown 2005).
Perspective for Other Demanding Climates
In this paper we have shown that there are both opportunities and challenges to stormwater (including snow) management using BGWI in cold climates. However, the BGWI concept is only applicable to regions where snow is a reoccurring weather condition that enables so-called white spaces. Consequently, for regions in warmer climates, the white dimension is not of interest. However, also in these regions, non-blue-green seasons are common. Thus, conditions that can be recognized by other dimensions of the BGI concept should be considered, for instance, yellow-brown spaces in regions with reoccurring dry seasons or drought [Fig. 1(b)] and gray-brown during winter in temperate climates [Fig. 1(c)]. By analyzing and identifying not only blue-green but all possible urban spaces, the expansion and application of the BGI concept has the potential to improve the delivery of year-round ESs and multiple benefits in cities globally.
Conclusions
At certain times of the year and in many regions BGI are neither blue nor green but rather white, yellow, or brown. In this paper, the potential for a range of ESs from white spaces is identified. Consequently, the theoretical framework developed for expanding BGI to BGWI is a promising concept. This approach allows for multiple benefits to be delivered by all three components of BGWI as well as establishing the interest of local stakeholders in adopting the approach within local urban planning. Though the technical functionality of BGI is well understood, its performance in cold climates (as BGWI) poses additional challenges and issues, especially when including multiple ESs. However, the expansion of the concept of BGI to BGWI will facilitate making urban services and public amenities functional across seasons and across a temperature range of to . However, this expansion also implies challenges, often in connection with regulations and economic and administrative responsibilities of snow- and stormwater management. To move forward and conceptualize BGI to include white spaces (as BGWI) specifically and other dimensions in demanding climates in general (e.g., blue-green-yellow/brown infrastructure), a better understanding of these challenges needs to be developed. We believe that if this is done, the possibility of contributing to achieving Sustainable Development Goals and other national sustainability requirements will be improved, not only in a Swedish context but wherever this conceptualization is established.
Data Availability Statement
Data that support the findings of this study are available from the corresponding author upon request.
Acknowledgments
This study was supported by the Swedish Research Council Formas (Grant 2021-01963). It was conducted within the frame of the DRIZZLE research center (Vinnova Grant 2016-05176). The authors thank Günther Leonhardt and Erik Hidman for their contribution to initial discussions on the topic of this paper.
References
Ashley, R. M., C. J. Digman, B. Horton, B. Gersonius, B. Smith, P. Shaffer, and A. Baylis. 2018. “Evaluating the longer term benefits of sustainable drainage.” Proc. Inst. Civ. Eng. Water Manage. 171 (2): 57–66. https://doi.org/10.1680/jwama.16.00118.
Brown, R. R. 2005. “Impediments to integrated urban stormwater management: The need for institutional reform.” Environ. Manage. 36 (3): 455–468. https://doi.org/10.1007/s00267-004-0217-4.
Davey, M. P., et al. 2019. “Snow algae communities in Antarctica: Metabolic and taxonomic composition.” New Phytol. 222 (3): 1242–1255. https://doi.org/10.1111/nph.15701.
Ellis, J. B., and L. Lundy. 2016. “Implementing sustainable drainage systems for urban surface water management within the regulatory framework in England and Wales.” J. Environ. Manage. 183 (Dec): 630–636. https://doi.org/10.1016/j.jenvman.2016.09.022.
Fletcher, T. D., et al. 2015. “SUDS, LID, BMPs, WSUD and more—The evolution and application of terminology surrounding urban drainage.” Urban Water J. 12 (7): 525–542. https://doi.org/10.1080/1573062X.2014.916314.
Gällivare Municipality (Gällivare kommun). 2016. “Grön-, blå- och vitstrukturplan Gällivare (Green, blue and white structure plan Gällivare).” Accessed April 26, 2019. http://www.gellivare.se/Nya-gallivare/Planarbete/Gron--bla--och-vitstrukturplan/.
German, J., G. Svensson, L. G. Gustafsson, and M. Vikström. 2003. “Modelling of temperature effects on removal efficiency and dissolved oxygen concentrations in stormwater ponds.” Water Sci. Technol. 48 (9): 145–154.
Johannessen, B. G., H. M. Hanslin, and T. M. Muthanna. 2017. “Green roof performance potential in cold and wet regions.” Ecol. Eng. 106 (Jun): 436–447. https://doi.org/10.1016/j.ecoleng.2017.06.011.
Kratky, H., Z. Li, Y. Chen, C. Wang, X. Li, and T. Yu. 2017. “A critical literature review of bioretention research for stormwater management in cold climate and future research recommendations.” Front. Environ. Sci. Eng. 11 (4): 16. https://doi.org/10.1007/s11783-017-0982-y.
Kuoppamäki, K. 2021. “Vegetated roofs for managing stormwater quantity in cold climate.” Ecol. Eng. 171 (21): 106388. https://doi.org/10.1016/j.ecoleng.2021.106388.
Lönnqvist, J., H. M. Hanslin, B. Gisvold Johannessen, T. M. Muthanna, M. Viklander, and G. Blecken. 2021. “Temperatures and precipitation affect vegetation dynamics on Scandinavian extensive green roofs.” Int. J. Biometeorol. 65 (Feb): 837–849. https://doi.org/10.1007/s00484-020-02060-2.
Lutz, D. A., and R. B. Howarth. 2015. “The price of snow: Albedo valuation and a case study for forest management.” Environ. Res. Lett. 10 (6): 064013. https://doi.org/10.1088/1748-9326/10/6/064013.
Marsalek, P. M., W. E. Watt, J. Marsalek, and B. C. Anderson. 2003. “Winter operation of an on-stream stormwater management pond.” Water Sci. Technol. 48 (9): 133–143. https://doi.org/10.2166/wst.2003.0510.
Moghadas, S., A. M. Gustafsson, P. Viklander, J. Marsalek, and M. Viklander. 2016. “Laboratory study of infiltration into two frozen engineered (sandy) soils recommended for bioretention.” Hydrol. Processes 30 (8): 1251–1264. https://doi.org/10.1002/hyp.10711.
Mortazavi, R., S. Attiya, and P. A. Ariya. 2019. “Diversity of metals and metal-interactive bacterial populations in different types of Arctic snow and frost flowers: Implications on snow freeze-melt processes in a changing climate.” Sci. Total Environ. 490 (Nov): 277–289. https://doi.org/10.1016/j.scitotenv.2019.06.350.
Muthanna, T. M., M. Viklander, G. T. Blecken, and S. T. Thorolfsson. 2007. “Snowmelt pollutant removal in bioretention areas.” Water Res. 41 (18): 4061–4072. https://doi.org/10.1016/j.watres.2007.05.040.
Nordell, B. 2015. “Using ice and snow in thermal energy storage systems.” In Woodhead publishing series in energy, Advances in thermal energy storage systems: Methods and applications, edited by Luisa F. Cabeza, 187–200. Sawston, UK: Woodhead Publishing.
Reinosdotter, K., and M. Viklander. 2006. “Handling of urban snow with regard to snow quality.” J. Environ. Eng. 132 (2): 271–278. https://doi.org/10.1061/(ASCE)0733-9372(2006)132:2(271).
Smith, A. C., et al. 2017. “How natural capital delivers ecosystem services: A typology derived from a systematic review.” Ecosyst. Serv. 26 (Aug): 111–126. https://doi.org/10.1016/j.ecoser.2017.06.006.
Søberg, L., G. T. Blecken, M. Viklander, and A. Hedström. 2014. “Metal uptake in three different plant species used for cold climate biofilter systems.” In Proc., 13th Int. Conf. on Urban Drainage. Kuching, Sarawak, Malaysia: Borneo Convention Centre.
Tahvonen, O. 2018. “Adapting bioretention construction details to local practices in Finland.” Sustainability 10 (2): 276. https://doi.org/10.3390/su10020276.
Tzoulas, K., K. Korpela, S. Venn, V. Yli-Pelkonen, A. Kaźmierczak, J. Niemela, and P. James. 2007. “Promoting ecosystem and human health in urban areas using green infrastructure: A literature review.” Landscape Urban Plann. 81 (Feb): 167–178. https://doi.org/10.1016/j.landurbplan.2007.02.001.
UK NEA. 2011. “UK national ecosystem assessment.” Accessed June 16, 2022. http://uknea.unep-wcmc.org/.
UN MEA. 2005. “Millennium ecosystem assessment.” Accessed June 16, 2022. https://www.millenniumassessment.org.
van der Meulen, E. S., L. C. Braat, and J. M. Brils. 2016. “Abiotic flows should be inherent part of ecosystem services classification.” Ecosyst. Serv. 19 (Mar): 1–5. https://doi.org/10.1016/j.ecoser.2016.03.007.
Vijayan, A., H. Österlund, J. Marsalek, and M. Viklander. 2019. “Laboratory melting of late-winter urban snow samples: The magnitude and dynamics of releases of heavy metals and PAHs.” Water Air Soil Pollut. 230 (Feb): 182. https://doi.org/10.1007/s11270-019-4201-2.
Wright, H. 2011. “Understanding green infrastructure: The development of a contested concept in England.” Local Environ. 16 (Sep): 1003–1019. https://doi.org/10.1080/13549839.2011.631993.
Information & Authors
Information
Published In
Copyright
This work is made available under the terms of the Creative Commons Attribution 4.0 International license, https://creativecommons.org/licenses/by/4.0/.
History
Received: Jan 25, 2022
Accepted: Apr 22, 2022
Published online: Aug 13, 2022
Published in print: Nov 1, 2022
Discussion open until: Jan 13, 2023
Authors
Metrics & Citations
Metrics
Citations
Download citation
If you have the appropriate software installed, you can download article citation data to the citation manager of your choice. Simply select your manager software from the list below and click Download.