Front matter for Total Maximum Daily Load Development and Implementation
Publication: Total Maximum Daily Load Development and Implementation: Models, Methods, and Resources
Abstract
Front matter pages come before the papers or chapters in a published work and include a title page, copyright information, and a table of contents. This publication's front matter also includes a list of other titles of interest, a foreword, a preface, acknowledgements, and an executive summary.
Other Titles of Interest
Concentrate Management in Desalination: Case Studies, 2nd Edition, edited by Berrin Tansel, Conrad G. Keyes Jr., Luzma F. Nava, and April J. Lander (ASCE/EWRI 2021). This report reviews the state-of-the-practice for managing the chemical concentrates resulting from the desalination process. (ISBN 978-0-7844-1569-6)
Computational Fluid Dynamics: Applications in Water, Wastewater, and Stormwater Treatment, edited by Xiaofeng Liu, Ph.D., P.E., and Jie Zhang, Ph.D (ASCE/EWRI 2019). This book provides an introduction, overview, and specific examples of computational fluid dynamics and their applications in the water, wastewater, and stormwater industry. (ISBN 978-0-7844-1531-3)
Statistical Analysis of Hydrologic Variables: Methods and Applications, edited by Ramesh S. V. Teegavarapu, Jose D. Salas, and Jery R. Stedinger (ASCE/EWRI 2019). This book provides a compilation of statistical analysis methods used to analyze and assess critical variables in the hydrological cycle. (ISBN 978-0-7844-1517-7)
Adventures in Managing Water: Real-World Engineering Experiences, edited by Pete Loucks and Laurel Sato (ASCE/EWRI 2019). This book provides valuable insight for students and professionals into real-world experiences of water engineers and other water management professionals. (ISBN 978-0-7844-1533-7)
Sustainable Water Resources Management, edited by Chandra S. P. Ojha, Rao Y. Surampalli, András Bárdossy, Tian C. Zhang, and Chih-Ming Kao (ASCE/EWRI 2017). This book presents the most current thinking on the environmental, social, and political dimensions of sustainably managing water supply at the local, regional, or basin levels. (ISBN 978-0-7844-1476-7)
Manuals and Reports on Engineering Practice
(As developed by the ASCE Technical Procedures Committee, July 1930, and revised March 1935, February 1962, and April 1982)
A manual or report in this series consists of an orderly presentation of facts on a particular subject, supplemented by an analysis of limitations and applications of these facts. It contains information useful to the average engineer in his or her everyday work, rather than findings that may be useful only occasionally or rarely. It is not in any sense a “standard,” however, nor is it so elementary or so conclusive as to provide a “rule of thumb” for non-engineers.
Furthermore, material in this series, in distinction from a paper (which expresses only one person's observations or opinions), is the work of a committee or group selected to assemble and express information on a specific topic. As often as practicable, the committee is under the direction of one or more of the Technical Divisions and Councils, and the product evolved has been subjected to review by the Executive Committee of the Division or Council. As a step in the process of this review, proposed manuscripts are often brought before the members of the Technical Divisions and Councils for comment, which may serve as the basis for improvement. When published, each manual shows the names of the committees by which it was compiled and indicates clearly the several processes through which it has passed in review, so that its merit may be definitely understood.
In February 1962 (and revised in April 1982), the Board of Direction voted to establish a series titled “Manuals and Reports on Engineering Practice” to include the manuals published and authorized to date, future Manuals of Professional Practice, and Reports on Engineering Practice. All such manual or report material of the Society would have been refereed in a manner approved by the Board Committee on Publications and would be bound, with applicable discussion, in books similar to past manuals. Numbering would be consecutive and would be a continuation of present manual numbers. In some cases of joint committee reports, bypassing of journal publications may be authorized.
A list of available Manuals of Practice can be found at https://www.asce.org/bookstore.
Foreword
The total maximum daily load or TMDL is a regulatory term associated with the United States Clean Water Act describing a plan for restoring the quality of impaired waters. The TMDL analysis establishes the maximum amount of a pollutant a waterbody can receive and still meet the applicable water quality standards. The term TMDL program is used to describe how the US government working with states, territories, and approved tribes (jurisdictions) manages the point and nonpoint sources of pollution to restore impaired waters. Implementing TMDLs is important to protect the environment and to reverse the adverse impacts of urbanization and agriculture on the waters of the United States.
Developing TMDLs is often supported by the applications of watershed and receiving water quality models that can provide rigor and clarity to the process. Concerns related to developing TMDLs include the following: the overwhelming numbers of models and analytical methods, potential for inappropriate selection of models, lack of uncertainty estimates, extensive data requirements, and infrequency of postimplementation assessments of performance. These concerns often lead to difficulties in the development, implementation, and sustainability of the TMDLs.
The ASCE EWRI Watershed Management Technical Committee (WMTC) formed the Task Committee on TMDL Analysis and Modeling in 2011 in response to the aforementioned concerns related to the use of models in TMDL development and implementation. The task committee consists of a diverse group of individuals representing important organizations that have a stake in the success of TMDL modeling. Committee members include practicing professionals, academics, and federal, state, and county experts in the TMDL process. The task committee recommended that an ASCE manual of practice be developed to assist practitioners carry out the steps of TMDL model selection, model input data collection and synthesis, model calibration, and independent testing of the model against known data or other observations to define uncertainty. Along the way, in generating this manual, the task committee published an ASCE EWRI report (book) in 2017 and, in 2019, published a collection of papers in the Journal of Hydrologic Engineering. In addition, the committee has held numerous planning and organizational meetings, both in-person and by conference and video calls. With the encouragement and guidance from the parent WMTC, task committee members have led TMDL workshops and technical sessions and have given presentations at ASCE EWRI and other conferences. This manual is the culmination of the combined efforts of the task committee.
The manual provides detailed descriptions, together with their relative strengths and limitations, of a large number of watershed models, receiving water quality models, and analytical methods/tools, some of which are widely used and others that have great potential for use in TMDL development and implementation. The manual highlights recent advances in TMDL analysis and modeling representing the state-of-the-art. However, the main purpose of the manual is to describe the state-of-the-practice as it relates to TMDL development and implementation, which includes the use of remote sensing and geographic information systems (GIS), standardized procedures for model calibration, validation, and testing; techniques for uncertainty analysis and protocols for defining the TMDL margin of safety. The manual provides example applications and sources (links) to previous TMDL study reports from around the United States. An online search tool is introduced that has the capability of performing detailed queries of the US Environmental Protection Agency (USEPA) ATTAINS database with respect to impairments, modeling techniques of interest, and constituents of concern.
The process leading to the development of this manual must have been both challenging and rewarding. I trust this manual will become a valuable reference and resource for those engaged in the practice of TMDL analysis and modeling, in that it provides a logical progression of steps for the development and implementation of TMDLs. Engineers, water quality professionals, regulators, and other water resource and watershed managers charged with the development and implementation of TMDLs should find this manual beneficial.
On a personal note, it has been a pleasure watching this group at work for the last several years. I want to commend the group—collectively and individually—for their dedication and hard work.
Donald K. Frevert, Ph.D., P.E., F.ASCE, Past-Chair
Watershed Council
Environmental and Water Resources Institute
Preface
The Total Maximum Daily Load or TMDL is a scientific and a regulatory term. Scientifically, it refers to the maximum amount of a pollutant a waterbody can receive and still meet the applicable water quality standards. In a regulatory context, TMDL is used in the United States to describe a document or plan of action to manage both point and nonpoint-source pollution of waterbodies. In this sense, a TMDL is a set of actions to restore clean water by examining water quality problems, identifying sources of pollutants, and specifying actions that create solutions. It is a written, quantitative plan and analysis for attaining and maintaining water quality standards in all seasons for a specific waterbody and pollutant. TMDLs are intended to be a part of a comprehensive watershed strategy to restore ambient water quality to meet its designated use (DU) and should involve collaboration with a wide variety of stakeholders.
As is the case with most natural systems, there are no experimental or direct methods to derive TMDL estimates. Developing a TMDL requires an analysis linking sources with loadings to an impairment for one or more pollutants, for which watershed and receiving-water quality modeling is vital. As found in the USEPA Assessment and Total Maximum Daily Load Tracking and Implementation System (ATTAINS), 76,127 TMDLs have been developed throughout the United States from 1975 through 2017 using various analytical or empirical methods or simple models, watershed models, and receiving water quality models. Models or methods are typically chosen by the individual TMDL developers as there exists no clear guidance or requirement regarding the analysis procedures or models to be used.
The TMDL Analysis and Modeling Task Committee of the ASCE EWRI was formed in October 2011 at the direction of the ASCE EWRI Watershed Management Technical Committee, which is under the ASCE EWRI Watershed Council, to address current concerns and advance the practices of analysis and modeling in TMDL development and implementation in terms of analysis technique and model selection, determining data requirements and processing, model calibration and validation, and uncertainty analysis and reporting. Members of the task committee are among the best available experts on TMDL analysis and modeling in the nation drawn from academia; federal, state, and local governments; and consulting firms. Membership has been stable over the period since the formation of the task committee with the exception of some leaving because of personal reasons and new members joining. The task committee met monthly via teleconferences and face-to-face at the annual ASCE EWRI World Environmental and Water Resources Congress during its existence and its members presented their work at the Congresses through workshops and technical sessions.
The task committee first reviewed the current practices of analysis and modeling in TMDLs and documented its findings in an ASCE EWRI book, Total Maximum Daily Load Analysis and Modeling: Assessment of the Practice in 2017. The members and subject expert authors involved in this publication were recognized. In addition, members of the committee completed a special collection of articles that were published under the title “Total Maximum Daily Load Analysis and Modeling: Assessment and Advancement” in the Journal of Hydrologic Engineering during the period 2018 to 2021. These articles provide state-of-the-art and state-of-the-practice overviews of sixteen relevant topics on TMDL analysis and modeling.
The ASCE-EWRI Task Committee on TMDL Analysis and Modeling then directed its attention to developing the current ASCE Manual of Practice titled, Total Maximum Daily Load Development and Implementation: Models, Methods, and Resources that reviews and evaluates current practices and potential future approaches to TMDL analysis and modeling in TMDL development and implementation. The primary goal of this manual was to support a more comprehensive and effective TMDL development and implementation that will prove to be beneficial to the public through eliminating current and future impairments to waterbodies and improving water quality for designated uses with confidence and certainty. The members of the task committee are responsible for authorship of this manual. Chapter authors are listed below under Contributing Authors and under the title of each chapter. All members of the task committee are also listed below with their affiliations, each contributed to this endeavor either by writing one or more chapters and/or participating in the review, and tasks of editing, revising, or providing constructive comments on various chapters. The book was organized and compiled by four editors listed on the cover page who are also long-serving and distinguished members of the task committee.
Thus, this manual on TMDL analysis and modeling for TMDL development and implementation resulted from research and deliberations of the task committee written in its 12 chapters by its members reflecting their collective expertise and experience. The 12 chapters consist of an introduction and a comprehensive coverage of eleven subtopics on TMDL modeling, methods, and resources needed to develop a TMDL and its implementation plan.
Deva K. Borah, Ph.D., P.E., F.ASCE, Life M.ASCE, Chair
TMDL Analysis and Modeling Task Committee
Environmental and Water Resources Institute
TMDL Analysis and Modeling Task Committee
Deva K. Borah, Ph.D., P.E., F.ASCE, Life M.ASCE, Chair
City of Chesapeake Department of Public Works
Saurav Kumar, Ph.D., P.E., M.ASCE, Vice Chair
Texas A&M AgriLife Research
Rosanna J. La Plante, P.E., F.ASCE, Secretary
Washington Suburban Sanitary Commission
G. Padmanabhan, Ph.D., P.E., F.ASCE, Life M. ASCE, Past Chair
North Dakota State University, Retired
William H. Frost, P.E., D.WRE, F.ASCE, Past Secretary
KCI Technologies, Inc.
Ebrahim Ahmadisharaf, Ph.D., M.ASCE
FAMU-FSU College of Engineering
Rene A. Camacho-Rincon, Ph.D., M.ASCE
Tetra Tech, Inc.
Xiaobo Chao, Ph.D., M.ASCE
University of Mississippi
Xing Fang, Ph.D., P.E., D.WRE, F.ASCE
Auburn University
Mohamed M. Hantush, Ph.D., M.ASCE
US Environmental Protection Agency
Sanaz Imen, Ph.D., P.E., M.ASCE
Stantec Engineering Services Company
Seshadri S. Iyer, Ph.D., P.E., F.ASCE
GKY & Associates, Inc.
Liping Jiang, Ph.D., A.M.ASCE
Montana Tech
Navaratnam Leelaruban, Ph.D., P.E., M.ASCE
HNTB Corporation
R. Craig Lott
Virginia Department of Environmental Quality
Steven C. McCutcheon, Ph.D., P.E., D.WRE (Ret.), F.ASCE
University of Georgia
Yusuf M. Mohamoud, Ph.D., P.E., M.ASCE
(Former member with coauthorships to chapters)
Natural Resources, Environment, and Technology Institute
Andrew Parker, M.ASCE
Tetra Tech, Inc.
Nigel W.T. Quinn, Ph.D., P.E., D.WRE, F.ASCE, Life M. ASCE
Lawrence Berkeley National Laboratory
Vamsi K. Sridharan, Ph.D., M.ASCE
University of California, Santa Cruz
Harry X. Zhang, Ph.D., P.E., M.ASCE
The Water Research Foundation
Zhonglong Zhang, Ph.D., P.E., M.ASCE
Portland State University
Blue-Ribbon Panel Reviewers
Latif Kalin, Ph.D.
Professor of Hydrology, Auburn University
Leslie Shoemaker, Ph.D.
President, Tetra Tech, Inc.
M. Todd Walter, Ph.D., M.ASCE
Professor of Biological and Environmental Engineering, Cornell University
Contributing Authors
Chapter 1: Introduction
Nigel W.T. Quinn, Steven C. McCutcheon, Vamsi K. Sridharan, Rosanna J. La Plante, Harry X. Zhang, Deva K. Borah, G. Padmanabhan
Chapter 2: Watershed Models
Deva K. Borah, Ebrahim Ahmadisharaf, G. Padmanabhan, Sanaz Imen, Harry X. Zhang, Yusuf M. Mohamoud, Zhonglong Zhang
Chapter 3: Receiving Water Quality Models
Rene A. Camacho-Rincon, Zhonglong Zhang, Xiaobo Chao
Chapter 4: Integrated Modeling Systems and Linked Models
Harry X. Zhang, Yusuf M. Mohamoud, Seshadri S. Iyer
Chapter 5: Simple Models and Methods
Harry X. Zhang, Nigel W.T. Quinn
Chapter 6: Critical Condition Determination for Total Maximum Daily Load Modeling
Harry X. Zhang, G. Padmanabhan
Chapter 7: Model Data, Geographical Information Systems, and Remote Sensing
Nigel W. T. Quinn, Saurav Kumar, Sanaz Imen, Vamsi K. Sridharan
Chapter 8: Model Calibration and Validation
Ebrahim Ahmadisharaf, Rene A. Camacho-Rincon, Harry X. Zhang, Mohamed M. Hantush, Yusuf M. Mohamoud
Chapter 9: Model Uncertainty Analysis and the Margin of Safety
Mohamed M. Hantush, Harry X. Zhang, Rene A. Camacho-Rincon, Ebrahim Ahmadisharaf, Yusuf M. Mohamoud
Chapter 10: USEPA Total Maximum Daily Load Report Archive and Report Search Tool
Nigel W.T. Quinn, Saurav Kumar, Rosanna J. La Plante
Chapter 11: Model Selection and Applications for Total Maximum Daily Load Development
Vamsi K. Sridharan, Steven C. McCutcheon, Nigel W. T. Quinn, Harry X. Zhang, Saurav Kumar, Ebrahim Ahmadisharaf, Xing Fang, Andrew Parker
Chapter 12: Modeling for Total Maximum Daily Load Implementation
William H. Frost, R. Craig Lott, Rosanna J. La Plante
Acknowledgments
This Manual of Practice on engineering practices of TMDL analysis and modeling in developing and implementing TMDLs was prepared by the ASCE/EWRI Task Committee on TMDL Analysis and Modeling under the Watershed Management Technical Committee of the Watershed Council, ASCE/EWRI, over a period of ten years (2011 to 2021) of focused and progressive efforts. Dr. G. Padmanabhan founded the task committee in 2011 as its chair, with Dr. Ashok Pandit as the vice chair and Mr. William Frost as secretary. Under their leadership, the task committee first published an ASCE EWRI book in 2017 titled Total Maximum Daily Load Analysis and Modeling: Assessment of the Practice. In 2017, the leadership of the task committee transitioned to Dr. Deva Borah as chair, Dr. Saurav Kumar as vice chair, and Ms. Rosanna La Plante as secretary. Under their leadership, the task committee published a special collection of articles on “TMDL Analysis and Modeling: Assessment and Advancement” in the Journal of Hydrologic Engineering during 2018 to 2021 (Guest editors: Dr. Deva Borah, Dr. G. Padmanabhan, and Dr. Saurav Kumar) and now this ASCE manual, edited by Dr. Harry Zhang, Dr. Nigel Quinn, Dr. Deva Borah, and Dr. G. Padmanabhan.
The editors thank all the members of the task committee for their contributions and/or serving as internal reviewers of the manual, in addition to their roles in achieving the task committee goals. The thoroughness of the reviews, editorial suggestions, and comments by Dr. Steven C. McCutcheon and Dr. Vamsi K. Sridharan are appreciated. The editors also thank the Blue-Ribbon Panel for reviewing the draft manual and providing helpful comments. The combined leadership, continued support and guidance of both the ASCE/EWRI Watershed Management Technical Committee and the Watershed Council were essential in helping the task committee achieve its stated goals.
In conclusion, we wish to express gratitude to the parent organizations of task committee members for providing staff time and committing other resources that helped to streamline the task committee endeavor to produce this manual.
Executive Summary
Background and Rationale
A total maximum daily load (TMDL) is the maximum allowable loading limit of a particular water quality constituent such as nutrients, sediment, temperature, salinity, pathogen counts, and others that can enter an impaired water system (receiving waterbody) identified under Section 303(d) of the Clean Water Act (CWA) of 1972, so that the applicable water quality standards can be met. In a regulatory context, the TMDL facilitates a plan of action to manage both point source (PS) and non-point-source (NPS) pollution. The minimum elements in a TMDL required by the USEPA's Guidelines for Reviewing TMDLs under Existing Regulations issued in 1992 are the following:
1.
Identification of waterbody, drainage area, pollutant(s) of concern, pollutant sources, and a reasonable load reduction possibility scenario;
2.
Development of applicable Water Quality Standards (WQS) or numeric water quality targets;
3.
Assessment of pollutant assimilative Load Capacity (LC) for the receiving water of concern;
4.
Computation of pollutant load allocations (LAs), waste load allocations (WLAs), and a margin of safety (MOS);
5.
Consideration of seasonal variations and critical conditions;
6.
Analysis of uncertainty and development of reasonable assurance guidance that pollutant PS/NPS load reductions are achievable;
7.
Development of an implementation plan with involvement of stakeholders;
8.
Formulation of an appropriate, cost-effective monitoring plan to track TMDL effectiveness; and
9.
Management of project outreach and public participation.
As is the case with most natural systems, there are no experimental or direct methods to derive a TMDL. Developing a TMDL is a systematic process that quantifies the extent of pollutant loading to a waterbody and develops a plan to address the water quality impairment for which the use of watershed and receiving water quality models is key. As documented in the USEPA Assessment and TMDL Tracking and Implementation System (ATTAINS), 76,127 TMDLs were developed across the United States during the period 1975 through 2017 employing a wide variety of analytical or empirical procedures/methods, simple models, watershed models, and receiving water quality models. The tools were chosen by the individual TMDL developers without the benefit of clear guidance or standardized protocols that might have guided the analysis procedures and led to better choice of model.
Although guidance manuals have been developed by USEPA for the purpose of pollutant load allocations, neither USEPA nor any state agency has developed specific guidance on TMDL analysis and modeling. The EWRI Watershed Management Technical Committee of the ASCE first chartered the Total Maximum Daily Load Analysis and Modeling Task Committee (TMDL-TC) in 2011 to explore the development of an ASCE Manual of Practice (MOP) on TMDL analysis and modeling for the development and implementation of TMDLs. After a careful review of current and past practices, the TMDL-TC confirmed the inadequacy of existing guidance for TMDL analysis and modeling. As stated in an ASCE book authored by the TMDL-TC in 2017, an ASCE MOP was both timely and critical to achieve the water quality objectives established by the CWA. In 2019, as an interim step, the TMDL-TC members published a special collection of papers in the ASCE Journal of Hydrologic Engineering that sought to define the scientific basis more clearly for TMDL modeling and describe the state-of-the-art. The MOP that followed the 2017 ASCE book and the special collection of associated technical papers relates the state-of-the-practice to the state of the art in TMDL analysis and modeling. It was not always possible to draw a clear distinction between these designations, given the rapid innovation in the embrace of technologies such as GIS and remote sensing and the realization that certain current practices may become obsolete in the not-too-distant future. The MOP addresses this and other relevant topics/subjects in each of the MOP chapters.
Summary of Chapters
The 12 chapters contained in the MOP are on TMDL analysis and modeling and other related topics needed in the development and implementation of TMDL arranged in an order corresponding to a typical TMDL development workflow to guide the readers. The chapters describe the various watershed and receiving water models; discuss the integration or linking of these models for complex waterbody systems; simple analytical and empirical procedures/methods and models; critical condition determinations; data types and sources; calibration, validation, and performance quantifications of the models; model uncertainty analysis and margin of safety quantification for TMDL developments; a database of USEPA-approved TMDL reports and a computer search tool to search those reports; a model selection protocol for TMDLs, the principal steps involved in modeling for TMDL development and implementation planning along with an implementation planning case study; and current analysis and modeling practices for TMDL implementation planning.
Brief summaries of the individual chapters are given below:
Chapter 1 Introduction: The chapter provides a brief history of the TMDL program and the overall TMDL process. It expands on the TMDL definition and rationale and purpose of the MOP that is summarized in the section above.
Chapter 2 Watershed Models: The chapter presents watershed models that are primarily needed and used in TMDL development and implementation. Fourteen prevalent watershed models, listed subsequently, were selected based on their documentation including user manuals, successful applications on various watersheds, evaluations, and acceptances found in the literature. In Chapter 2, these 14 watershed models are further evaluated to determine their capabilities suitable for TMDL development and implementation.
1.
Agricultural Nonpoint Source (AGNPS) and Annualized AGNPS (AnnAGNPS),
2.
Areal Nonpoint Source Watershed Environment Response Simulation (ANSWERS),
3.
Dynamic Watershed Simulation Model (DWSM),
4.
Gridded Surface and Subsurface Hydrologic Analysis (GSSHA),
5.
Generalized Watershed Loading Function (GWLF),
6.
Hydrologic Engineering Center–Hydrologic Modeling System (HEC–HMS),
7.
Hydrological Simulation Program–Fortran (HSPF),
8.
Kinematic Runoff and Erosion (KINEROS),
9.
Loading Simulation Program in C++ (LSPC)–HSPF based,
10.
Mike Systém Hydrologique Européen (MIKE SHE),
11.
Soil and Water Assessment Tool (SWAT),
12.
Storm Water Management Model (SWMM),
13.
Watershed Assessment Model (WAM), and
14.
Watershed Analysis Risk Management Framework (WARMF).
Brief descriptions of these watershed models, including sources, capabilities, and applicability, are presented in Chapter 2. General information such as intended watershed (agriculture, urban or mixed), simulation type (event, continuous or both), simulated variables, uncertainty analysis, graphical user interface, and availability of the 14 models is presented in a comprehensive tabular form to compare the attributes of the models. The theoretical basis for the various hydrologic and water quality processes (phases or components) for each of the 14 models are tabulated for easy comparison of the models. Looking at the theoretical basis of models is important as it suggests expected performance and accuracy and dictate model features such as structure, input data, and model parameters. Among these, the water routing procedures derived from approximations of the St. Venant shallow water equations are the primary drivers of the models, based on which the models are compared and ranked on pictorial graphs based on the level of physical basis or accuracy against complexity. For example, the GSSHA and MIKE SHE models rank high on the simulation of overland flows and the SWMM ranks high in channel or pipe flow routings using the most accurate physical process–based equations—also being the most numerically complex. The GWLF model ranks at the bottom with respect to both flow simulation methodologies. The remainder of the models falls in between the two end members, although the DWSM model is the most computationally efficient among the kinematic wave models. Notable strengths and limitations of the watershed models for TMDL development and implementation are tabulated. This valuable compilation of information on the subject models is unique and should aid comparisons of relative model reliability and help guide selections for TMDL and other environmental applications.
Of the 14 watershed models presented in Chapter 2, applications of 12 (HSPF, LSPC, GWLF, SWAT, SWMM, WARMF, AGNPS, ANSWERS, HEC–HMS, KINEROS, WAM, and MIKE SHE) can be found in TMDL studies. However, caution should be exercised to their appropriateness for all applications - misuses of models are sometimes found in past TMDL study reports. Although the DWSM and GSSHA models have not been used in TMDL applications, these two models have been evaluated and used in other watershed management and best management practices (BMP) evaluation studies and, therefore, would be suitable for future TMDL development and implementation studies.
Chapter 2 outlines future research on watershed modeling. This research should include further comparisons of models performance based on other key aspects such as simulation capabilities of processes, uncertainty analyses, required resources, and ability to characterize pollutant loading from complex watersheds. Robust physically based algorithms, uncertainty analysis capabilities, and improvements in the facility of use of remotely sensed and high-resolution data for model development are recommended to be part of the future advancement in modeling technology, application, and solution rigor.
Chapter 3 Receiving Water Quality Models: The chapter describes the following 11 receiving water quality models that were previously used in TMDL development and implementation planning:
1.
CE-QUAL-ICM Three-Dimensional Eutrophication Model,
2.
Water Analysis Simulation Program (WASP),
3.
Environmental Fluid Dynamics Code (EFDC),
4.
CE-QUAL-W2 Two-Dimensional Longitudinal–Vertical Hydrodynamic and Water Quality Model,
5.
Hydrologic Engineering Center River Analysis System (HEC–RAS),
6.
Center for Computational Hydroscience and Engineering Model (CCHE-1D/2D/3D),
7.
Georgia Environmental Protection Division River Model (EPD-RIV1),
8.
QUAL2K River and Stream Water Quality Model,
9.
MINTEQA2/Visual MINTEQ Geochemical Equilibrium Specification for Dilute Aqueous Systems,
10.
One-dimensional Transport with Equilibrium Chemistry Model (OTEQ), and
11.
MIKE11-ECOLAB River Hydraulics and Sediment Transport with Water Quality Simulations Model.
The sources, simulation capabilities, and applications of each of the 11 receiving water quality models are presented and briefly described in Chapter 3. Availability of these models in the public domain and specific capabilities of each of the models, such as 1D, 2D, or 3D hydrodynamic routings; types of modeling systems (rivers, lakes, reservoirs, or estuaries); and simulating pollutants (temperature, salinity, dissolved oxygen, carbon, nitrogen, phosphorous, algae, sediment, pathogen, toxics, and metals) are summarized in a table/matrix facilitating comparisons and the best selections of the models for applications in various TMDL studies (analysis). Like watershed modeling, receiving water quality modeling has also been researched and developed for over half a century. Thus, in this instance, the state-of-the-practice has caught up with the state-of-the-art. Chapter 3 serves as a valuable resource to understand the capabilities of the 11 models and match those with the conditions of the impairments and the impaired waterbodies for selecting the best model(s) for TMDL development and implementation.
Chapter 4 Integrated Modeling Systems and Linked Models: In this chapter, integrated modeling systems and linked models are described with examples. Linking of different models is needed to represent complex natural systems such as watersheds and their estuaries when such systems cannot be represented by a single model. Linkages can be static or dynamic. A static linkage takes output from one model and uses it as input to a second model. A dynamic linkage can be bidirectional, where information from each time step transfers back and forth between the models and affects simulations of all. Integrated modeling systems and linked models can satisfy multiple model application criteria because they include the capabilities of multiple models, provide various software tools, and include data and analysis support. Chapter 4 presents two widely used integrated modeling systems, briefly summarized in the following two paragraphs, along with a listing or brief descriptions of example applications in TMDL studies. Also, as summarized subsequently, three example applications of linked models are presented in Chapter 4 along with an important discussion on the performance evaluations of integrated modeling systems and linked models in TMDL applications.
1.
BASINS: The Better Assessment Science Integrating point and Nonpoint Sources (BASINS) System developed by USEPA, initially in 1996 and continually updated with the latest BASINS 4.5 release in 2019, is an integrated modeling system using various components and utilities and currently supporting seven models, AQUATOX, GWLF-E, HSPF, PLOAD, SWAT, SWMM, and WASP, for TMDL development and implementation. The origin and history of BASINS, its various components and utilities, and brief descriptions and one TMDL application example of each of the supporting models are presented and/or listed in Chapter 4.
2.
WMS: The Watershed Modeling System (WMS) of the US Army Corps of Engineers is a comprehensive graphical modeling environment for all phases of watershed hydrology and hydraulics, which has also been used to support TMDL development. The WMS supports hydrologic modeling with the HEC-1/HEC–HMS, TR-20, TR-55, Rational Method, National Flood Frequency Program (NFF), HSPF, and GSSHA. It also supports hydraulic models such as the HEC–RAS and CE-QUAL-W2. A few example applications of the WMS are briefly described in Chapter 4.
In addition to citing a few applications of linked models, Chapter 4 describes the following three example applications of linked models in TMDL development and implementation:
1.
Chesapeake Bay TMDL in the Mid-Atlantic United States: Chesapeake Bay TMDL was developed linking the HSPF, modeling its 64,000-sq mi watershed, with CMAQ, a Community Multi-Scale Air Quality model, simulating atmospheric deposition of nutrients, and with the Water Quality and Sediment Transport Model (WQSTM) for estuaries, simulating hydrodynamics, sediment and nutrient transport, and eutrophication processes inside the Bay for allocating total nitrogen, total phosphorus, and sediment loads from the sources to reduce the size of the oxygen-depleted zones in the Bay and for restoring its living resources.
2.
The Saugahatchee Creek Watershed in Alabama: The Alabama Department of Environmental Management (ADEM) identified two segments within the Saugahatchee Creek watershed of the Lower Tallapoosa River basin as being impaired for nutrients and organic enrichment/dissolved oxygen. The LSPC, EFDC, and WASP models were linked to simulate, respectively, runoff- and flow-generated sediment and phosphorus loadings from nonpoint sources, hydrodynamics, and water quality in the impaired waterbodies in an effort to determine load allocations from the sources and meet the water quality standards of the impaired waterbodies for the designated uses.
3.
The Lynnhaven River in the Atlantic coast of Virginia: The HSPF from the BASINS was linked with the UnTRIM and CE-QUAL-ICM to simulate, respectively, runoff-generated flow and pollutant loadings from the Lynnhaven River watershed, hydrodynamics in this tidal estuary, and water quality in this estuarine river in an effort to determine load allocations from the sources and meet the water quality standards of the Lynnhaven River that was impaired with fecal coliform, nitrogen, phosphorus, and sediment.
Chapter 5 Simple Models and Methods: The chapter presents simple models and methods that are used to support assessment of the relative significance of different pollutant sources and to guide decisions for management plans. These models and methods are typically derived from empirical relationships between the physiographic characteristics of the watershed and pollutant exports and are often used when data limitations and budget and time constraints preclude using more detailed approaches. These approaches fit the needs of stakeholders tasked with implementing TMDLs where the focus is developing an understanding of the sources and quantification of pollutants causing impairments in a waterbody and determining how best to reduce the loads from prioritized pollutant sources that had been identified in the TMDL from previous monitoring, modeling, and analysis. The following is a list of 13 simple models and methods available to simulate watersheds (10 models/methods) and receiving waterbodies (three models/methods) that are discussed in Chapter 5:
A.
Simple Watershed Models and Methods
1.
Simple mass balance,
2.
Simple method to estimate urban stormwater loads,
3.
Watershed Treatment Model (WTM),
4.
Spreadsheet Tool for Estimating Pollutant Loads (STEPL),
5.
Revised Universal Soil Loss Equation 2 (RUSLE2),
6.
Load Estimator (LOADEST),
7.
Spatially Referenced Regressions on Watershed Attributes (SPARROW),
8.
Long-Term Hydrologic Impact Analysis (L-THIA),
9.
Simple Transient Mass Balance Models,
a.
Wetland Management Simulator (WETMANSIM)
b.
San Joaquin River Input–Output Model (SJRIO)
10.
Geographical Information System (GIS) Workflow Models.
b.
Simple Receiving Water Models and Methods
1.
BATHTUB,
2.
Stream Segment Temperature Model (SSTEMP), and
3.
Load Duration Curve (LDC).
The aforementioned procedures and models are limited in many ways and, therefore, a knowledge of these limitations is critical in the successful application of the procedures and the models. One major advantage is that these tools can provide a rapid means of identifying critical loading areas with minimal effort and data requirements. A major disadvantage is that only gross estimates of nutrient loads can be provided and are of limited value for determining loads on a seasonal or finer timescale. Another disadvantage is that these techniques may be too generalized and of limited use for evaluating the effectiveness of various BMPs. However, a strategy that starts with simple models in the initial phases and then progresses to more complex frameworks as additional data are collected, and as appropriate remedial measures are assessed, can be successful and cost-effective. Thus, simple models and methods can play an important role in TMDL development and evaluation as model selection invariably involves trade-offs involving model complexity, required reliability, cost, and time.
Chapter 6 Critical Condition Determination for Total Maximum Daily Load Modeling: In this chapter, critical condition determination in developing a TMDL and the appropriate model or methodologies are discussed. According to the Clean Water Act, the USEPA TMDL program requires a consideration of the critical conditions for streamflow, loading, and water quality parameters for the development of TMDLs. The intent is to ensure that the water quality of an impaired waterbody is protected during times when it is most vulnerable, such as during low- or high-flow periods. The following four methods have been used in critical condition modeling and analysis in TMDL development and implementation (examples are provided in this chapter):
1.
Steady-state models for analyzing impairment under constant flows,
2.
Dynamic continuous simulation models for analyzing impairment under variable flows,
3.
Statistical-based Load-Duration Curves (LDC) for all possible flow conditions, and
4.
Event-based critical flow storm (CFS) with risk-based approach to combined conditions.
Low-flow analyses are performed using steady-state models. For TMDLs related to impaired waterbodies affected by pollutants from both point and nonpoint sources, a dynamic continuous model is commonly used along with the selection of a representative period to account for the varying climatic and hydrologic conditions in a watershed (e.g., to cover dry, wet, and average years of precipitation). The load-duration curve method is a simple screening tool for problem characterization as part of TMDL development. The event-based critical flow-storm method is essentially a risk-based approach that explicitly addresses critical condition as a combination of streamflow, the magnitude of a storm event, and initial watershed conditions. Decision-making authorities could substantially benefit from information on risk and the associated probabilities of various combinations of rainfall, river flow conditions, and the pollutant loads generated under these combinations.
Chapter 7 Model Data, Geographic Information Systems, and Remote Sensing: The chapter outlines the data requirements in modeling for TMDL development and describes the geographic information system (GIS) and remote sensing (RS) technologies available for the acquisition of high-resolution spatial and temporal data needed to set up, initialize, and run detailed watershed models. Data requirements for a TMDL model setup are tabulated; also tabulated are data requirements for running the TMDL models. An overview of the GIS and an up-to-date evolution of the RS are presented. Also discussed are the specific data needs for certain TMDL applications and how the RS platforms can be matched to these specific data requirements. RS has added another dimension to measuring and retrieving hydrologic and atmospheric data for water quality and hydrologic modeling. Along with the advancements in RS technology, acquisition, and processing of large amounts of information are being managed using robust and easy-to-use GIS software both in the commercial and in the public domain. ArcGIS and other GISs are ubiquitous and have evolved to a point where these tools are extensively used in watershed and impaired waterbody modeling. Chapter 7 provides an overview of the more common GIS and RS technologies used in hydrologic, water quality, and TMDL modeling applications.
Chapter 8 Model Calibration and Validation: The chapter presents and discusses state-of-the-art approaches in model calibration and validation as found in the literature as a guide for the calibration and validation of models applied in TMDL development and implementation. The chapter focuses on watershed models, although the same are applicable to receiving water models as well. Model data requirements and sources of the model data presented in Chapter 7 are further expanded and refined in this chapter by tabulating various types of data and sources and providing website links of the well-maintained data sources. Discussions on data management and guidance on pre-calibration; calibration, including manual calibration and auto-calibration; and validation are presented. The principal parameters requiring calibration in the 14 watershed models presented in Chapter 2 (a few exceptions) are tabulated separately for hydrologic and water quality simulations, which are valuable resources to modelers. Measuring model performance or calibration success is discussed, including a tabulation of various formulas found in the literature for the goodness-of-fit measures of watershed and receiving water quality model predictions. Acceptance criteria of some of the measuring formulas or methods are also tabulated.
Chapter 9 Model Uncertainty Analysis and Margin of Safety: The chapter presents and discusses uncertainties in modeling, and accounting these in a Clean Water Act mandated margin of safety (MOS) in TMDL development and implementation, along with several estimation methods, including explicit, implicit, and risk-based MOS methods. In addition, a survey of sampled 17 TMDL reports is presented in a table compiling the TMDL case study, the state in which they are located, impairment (pollutants), the TMDL model or method used, and the MOS estimation method used. A comprehensive review of uncertainty analysis methods, including MOS estimations, are presented and discussed, and practitioners may find them helpful in TMDL development and implementation. Uncertainty analysis methods applied in 19 water quality modeling studies are tabulated listing the methods and the models used, the impairments (pollutants), and study references, a valuable resource for modelers or practitioners. Detailed descriptions and some of the equations of some of those methods are presented. The methods are (1) first-order variance analysis, (2) Monte Carlo method, (3) Bayesian Monte Carlo analysis, (4) the generalized likelihood uncertainty estimation (GLUE) method, (5) the Markov Chain Monte Carlo method, and (6) Kalman Filtering.
Chapter 10 USEPA Total Maximum Daily Load Report Archive and Report Search Tool: The chapter briefly describes the USEPA TMDL report archive database and online system, called ATTAINS (Assessment and Total Maximum Daily Load Tracking and Implementation System), and presents a new online tool developed for searching TMDL reports for certain search categories such as specific pollutants (impairments), models, or others. Previously published TMDL reports may serve as helpful guides in developing new TMDLs. A web-based software (tool) called the “TMDL Report Selection” (TRS) tool was developed as part of the TMDL Analysis and Modeling Task Committee's efforts to search and analyze published TMDL reports found in ATTAINS. The TRS tool is a state-of-the-art search tool that promotes greater use of information found in reports of USEPA-approved TMDL projects in the USEPA archive ATTAINS.
In Chapter 10, the TRS tool and its application examples provide statistics from TMDL reports published during the period 1975 to 2017. These statistics include (1) the number of reports published in each of the 55 states; (2) number of reports published during each of the years 1975 to 2017; (3) number of reports using each of the selected methods or models including watershed models, receiving water quality models, and simple models; (4) number of reports addressing each of the impairments; and (5) number of reports using each of the selected methods or models that address each of the selected impairments. Guidance on using the TRS tool is also provided.
Chapter 11 Model Selection and Applications for Total Maximum Daily Load Development: In this chapter, a TMDL model selection protocol is presented with descriptions of its basis and various considerations (technical, management, and stakeholder and expert engagements) in the development process and summarizing these in an easy-to-read flowchart. The chapter also describes the process of applying models in the development and implementation of TMDLs and briefly describes a case study for evaluating TMDL load reduction scenarios in the form of an appendix in this chapter. It first lists the types of models used to develop TMDLs and then presents a holistic approach and a protocol to select a practical and dependable model for TMDL development and implementation. In this protocol, rigorous hypothesis testing of the selected model is emphasized, particularly if the model has not already been rigorously peer reviewed. The holistic protocol covers all aspects of the model application, from conceptualization and stakeholder collaboration to implementation planning and outreach. These considerations are translated into technical and management selection criteria and synoptic data requirements. Model performance evaluation, calibration, validation, and uncertainty estimation are all model application steps that influence selection of the model as described in Chapter 11. It also includes the modeling procedures necessary to support TMDL determination, allocation, and implementation planning. Looking into these steps ensures that the selected TMDL model is appropriate and likely to be successful and approved by the USEPA, which also issues National Pollution Discharge Elimination System (NPDES) permits. Other federal, state, and local agencies and stakeholders have roles in applying best management practices and other controls and overseeing the collection of synoptic data for calibration, validation, and determining model reliability. Finally, a discussion on the current state of the TMDL modeling practices and potential future innovations (developments) is presented.
Chapter 12 Modeling for Total Maximum Daily Load Implementation: The chapter discusses state-of-the-practice on the uses of models in TMDL implementation planning. Ideally, the same model used in TMDL development can also be used for TMDL implementation and related assessment; however, this is not always the case. Communities, organizations, agencies, and other stakeholders implementing a TMDL typically seek the most reliable and importantly the most cost-effective methods to achieve the load reductions required. They invariably intend to compare different scenarios that provide trade-offs among measures such as higher load reduction, lower cost, lower maintenance, available land, or easier implementation. In making these comparisons, the use of an easily understood and reliable model to estimate the load reduction from different planning scenarios is an important element of decision-making for TMDL implementation.
Chapter 12 discusses the uses of the following few watershed-specific models:
1.
Chesapeake Assessment Scenario Tool (CAST) for TMDL implementation with the Chesapeake Bay watershed;
2.
ArcView Generalized Watershed Loading Function (AVGWLF) based on the GWLF model (Chapter 2) and MapShed for Pennsylvania watersheds;
3.
Source Loading and Management for Windows (WinSLAMM) for Wisconsin watersheds; and
4.
P-8 Urban Catchment Model for Wisconsin watersheds.
Chapter 12 also discusses two spreadsheet analysis packages:
5.
Watershed Treatment Model (WTM) (Chapter 5), and
6.
Spreadsheet Tool for Estimating Pollutant Load (STEPL) (Chapter 5).
Critical comparative information (structure, capabilities, and treatment practices) of the aforementioned six models are tabulated for comparisons. Finally, a brief description of custom modeling using GIS geodatabases is presented with the example of the integrated modeling tool for scenario development for the District of Columbia. From an overall implementation perspective, Chapter 12 summarizes a number of ways by which TMDL implementation can be carried out with a better probability of success, including an adaptive management approach for complex TMDLs.
Concluding Remarks
This manual describes the state-of-the-practice and provides guidance to practitioners in selecting analytical tools and models in the development of a TMDL and its implementation plan. It includes detailed descriptions of a variety of watershed and receiving water quality models that can be used, highlighting recent advances in TMDL analysis and modeling.
This manual comprising 12 chapters is expected to serve as a useful resource to engineers, practitioners, regulators, and other water resource and watershed managers charged with developing and implementing TMDLs. The manual will also serve as a useful resource to planners, environmental scientists, students, educators, the concerned citizens, watershed stakeholders, and the public.
All chapters are based on state-of-the-art reviews of the respective topics, except Chapters 10 and 11, which introduce a state-of-the-art TMDL report search tool in the ATTAINS database and a state-of-the-art TMDL model selection protocol. All chapters serve as useful resources or tools in developing and implementing reliable and successful TMDLs.
As more TMDL studies result in implementation, the use of models for management planning and alternate analysis is increasing; for example, explicit incorporation of BMPs and low-impact development (LID) in the watershed and water quality models has advanced in recent years. The advent of machine learning techniques and other statistical data science technologies can assist communities and watershed stakeholders in achieving TMDL pollutant load reduction goals in a more sustainable and cost-efficient manner. Thus, this Manual of Practice expects to become a living document and needs to be updated in the future to account for potential advances in the practice of TMDL analysis and modeling for the development and implementation of TMDLs.
Information & Authors
Information
Published In
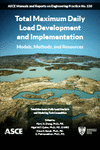
Total Maximum Daily Load Development and Implementation: Models, Methods, and Resources
Pages: i - xxxix
Editors: Harry X. Zhang, Ph.D., Nigel W.T. Quinn, Ph.D. https://orcid.org/0000-0003-3333-4763, Deva K. Borah, Ph.D. https://orcid.org/0000-0002-2107-9390, and G. Padmanabhan, Ph.D. https://orcid.org/0000-0002-3209-1379
ISBN (Print): 978-0-7844-1594-8
ISBN (Online): 978-0-7844-8382-4
Copyright
© 2022 American Society of Civil Engineers.
History
Published online: Feb 24, 2022
Published in print: Mar 1, 2022
Authors
Metrics & Citations
Metrics
Citations
Download citation
If you have the appropriate software installed, you can download article citation data to the citation manager of your choice. Simply select your manager software from the list below and click Download.