Characteristics of Concrete Corroded by Landfill Leachate in Extremely Cold Regions
Publication: Journal of Cold Regions Engineering
Volume 38, Issue 4
Abstract
In extremely cold regions with frequent extreme weather conditions, coupled factors make concrete corrosion by garbage landfill leachate become more complicated. Although previous studies have characterized the effects of individual factors, the mechanisms of coupled effects under specific conditions require further research. Therefore, this study analyzed the individual and coupled effects of landfill leachate, salt solution, and freeze–thaw cycles. The corrosion mechanism of landfill leachate was explained by analyzing the mechanical properties, surface morphology, and microstructure of the concrete. The individual factor tests showed that landfill leachate affected the performance of concrete more significantly than salt solution in the short term. In the immersion tests, the strength of concrete specimens corroded by landfill leachate was always minimized throughout the process, and the compressive and flexural strengths of concrete in landfill leachate decreased by 6.40% and 7.83%, respectively. In the coupled tests, freeze–thaw cycles amplified the effect of landfill leachate on concrete, exhibiting more severe damage than that with only landfill leachate. After four test cycles, the compressive strength of the concrete in the coupled test group decreased by 22.5%, 19.7%, and 31.3% and the flexural strength decreased by 40.2%, 26.7%, and 46.3%, respectively. Through surface morphology and microstructure analyses, we found that the inorganic salt ions in the landfill leachate reacted to form expansive ettringite and gypsum, which initially optimized the internal structure of the concrete but caused severe damage in the later stage. Concrete corrosion by landfill leachate was significantly enhanced under the influence of freeze–thaw cycles. The experimental results of this study provide basic data and theoretical support for designing and protecting concrete buildings in extremely cold environments.
Practical Applications
The deterioration of concrete buildings in landfills by landfill leachate corrosion has received widespread attention, and this problem is pronounced in cold regions. This study selected landfill leachate, salt solution, and freeze–thaw cycles as the corrosion conditions for the concrete corrosion test under one-factor conditions. At the same time, landfill leachate and salt solution were coupled with freeze–thaw cycles for the concrete corrosion test. The severity of concrete damage was compared by the changes in mechanical properties and microscopic morphology of the concrete during the test. The results of the one-factor corrosion test showed that the landfill leachate would cause more damage to the concrete in the short term. Meanwhile, the coupled-factor corrosion test results showed that freeze–thaw cycles amplify concrete corrosion by landfill leachate, resulting in a significant reduction in the mechanical properties of concrete and damage to the internal structure. In addition, the study characterized the mechanism of action of landfill leachate corrosion on concrete. This could also provide theoretical support for constructing and protecting concrete structures in landfills in cold regions.
Introduction
Landfills in extremely cold regions are subject to complex corrosion conditions and are often affected by factors such as landfill leachate, freeze–thaw cycles, salt corrosion, and microbial corrosion (Chen et al. 2023; Liu et al. 2009; Zhou et al. 2021; Nica et al. 2000), resulting in much higher corrosion rates of internal concrete structures than for traditional landfills. The landfill leachate produced by the complex environmental factors and microbial actions of garbage stacked in landfills (Miller and Emge 1997) contains various microorganisms, high organic matter content, and corrosive ions (Amor et al. 2015; Thornton and Blanc 1973; Gong et al. 2023; Cheng et al. 2022) that significantly damage the internal structure of concrete, posing a serious threat to the durability and service life of concrete structures (Wan and Wan 2011; Wu et al. 2021; Li et al. 2019; Park et al. 2021).
This problem has received increasing attention from researchers, and several studies have been conducted on the impact of landfill leachate on concrete. Feng (2022) used nanoindentation and uniaxial compression tests to study changes in the internal structure of concrete after leaching corrosion, which ultimately led to a decrease in its elastic modulus and compressive strength. Wan and Wan (2011) analyzed the chemical composition of landfill leachate and further studied the physical properties and microscopic structure of concrete specimens, concluding that salt ions, heavy metals, and microorganisms in landfill leachate delayed the hardening process of concrete and reduced its mechanical properties. Yang et al. (2005) analyzed and surveyed the chemical properties of landfill leachate and, based on its corrosive substances, determined its corrosion level on concrete as strong corrosion. Anand and Palani (2022) used ecotoxicity tests to assess the toxicity and pollution potential of landfill leachate, analyzed its chemical composition, and interpreted its potential negative impacts. Wdowczyk and Szymańska-Pulikowska (2021) conducted on-site multidimensional statistics of a landfill site, detected relevant toxicity pollution indicators, and established impact tests to comprehensively study and analyze the properties of landfill leachate. Rustamov et al. (2021) found that the compressive and flexural strengths of concrete were significantly reduced after repeated freeze–thaw cycles. Zhang et al. (2017) used numerical simulation methods to show that cracks in concrete significantly increased the diffusion rate of chloride ions, causing adverse effects on the interior of concrete. Osman et al. (2021) and Dubravka et al. (2010) experimentally found that microorganisms effectively changed the appearance and internal structure of concrete, causing severe damage in harsh environments; they summarized the macroscopic corrosion evolution rules of concrete and the protective methods of concrete against microbial corrosion, respectively.
Based on previous studies, landfill leachate, freeze–thaw cycles, high salt concentrations, and microorganisms all impact concrete to a certain extent (Wang et al. 2020; Lines et al. 2021; Li and Huang 2021; Ren et al. 2018). However, owing to environmental differences, the mechanisms underlying these influencing factors vary significantly under specific conditions. Under complex conditions, we must consider the coupling effect of multiple factors on the durability of concrete to establish relevant research mechanisms more accurately (Xiao 2010). Many scholars have begun to focus on these issues. Diao et al. (2011) found that under the alternating action of freezing and thawing and mixed salt solutions, the compressive strength and modulus of elasticity of concrete decrease with increasing freezing and thawing and increase the compressive strain corresponding to the maximum compressive stress. Chen et al. (2021) studied the corrosion of concrete under different salt solution concentrations and types under freeze–thaw cycle conditions, analyzed the degree and mechanism of concrete damage, and established a mathematical model to describe the damage characteristics of concrete under coupled corrosion conditions. Li et al. (2016) proposed a dispersion model for chloride ions in concrete under freeze–thaw cycle conditions, further analyzed the factors affecting chloride ion diffusion, and found that concrete structures were severely damaged after being subjected to chloride ion corrosion and freeze–thaw cycles.
Currently, the need to identify the corrosion mechanism of landfill leachate on concrete in cold regions is urgent to meet future requirements and establish relevant measures for the protection of concrete structures. Therefore, the purpose of this study was to determine the effect of a single corrosion condition on concrete and to analyze its corrosion mechanism. Then, based on previous research, two severe corrosion conditions were selected for coupling, and a comparative study was conducted to explore the corrosion characteristics of landfill leachate on concrete in cold regions from macro- and microperspectives.
Experimental Section
Materials
The landfill leachate used in this experiment was selected from a representative sample collected on-site at a landfill in the Xiangfang District, Harbin. The landfill has been in place for over 5 years and mainly handles household waste. The samples required for the experiment were obtained from the conditioning tank of the plant. Its physical parameters are presented in Table 1. Once sealed in barrels, the landfill leachate was transported to a low-temperature laboratory for use. Experiments were conducted to test and analyze the water quality of aged landfill leachate (landfilled for more than 5 years) and fresh landfill leachate (landfilled for less than 5 years). Testing revealed that the ion content in the aged landfill leachate was much higher than that in the fresh landfill leachate. Therefore, the aged landfill leachate was selected as the corrosion solution for this experiment.
Serial number | Pollutant | Fresh landfill leachate | Aged landfill leachate |
---|---|---|---|
1 | pH | 7.92 | 7.65 |
2 | COD (mg/L) | 14,631 | 9,259 |
3 | Ammonia nitrogen (mg/L) | 2,618 | 3,804 |
4 | Total nitrogen (mg/L) | 6,539 | 4,630 |
5 | Total phosphorus (mg/L) | 170.8 | 70 |
6 | Suspended solids (SS) | 550 | 400 |
7 | Fluoride ions (mg/L) | 0.85 | 11.7 |
8 | Chlorine ions (mg/L) | 3,676.5 | 4,607.9 |
9 | Sulfate ions (mg/L) | 768.5 | 6,759.9 |
The cementitious material used in this test was Swan PO 42.5 grade composite silicate cement, and the fine and coarse aggregates were natural medium-coarse river sand and 5–15-mm continuous graded stone, respectively. Laboratory tap water was also used. The cement:water:fine aggregate:coarse aggregate ratio of the concrete was 1.00:0.48:1.71:3.12. Owing to the special nature of the test, the concrete test blocks used were 40 × 40 × 160 mm3 prisms and 40 × 40 × 40 mm3 cubes, prepared according to the standard process of production. The standard curing was completed after 28 days. The preparation process is shown in Fig. 1.
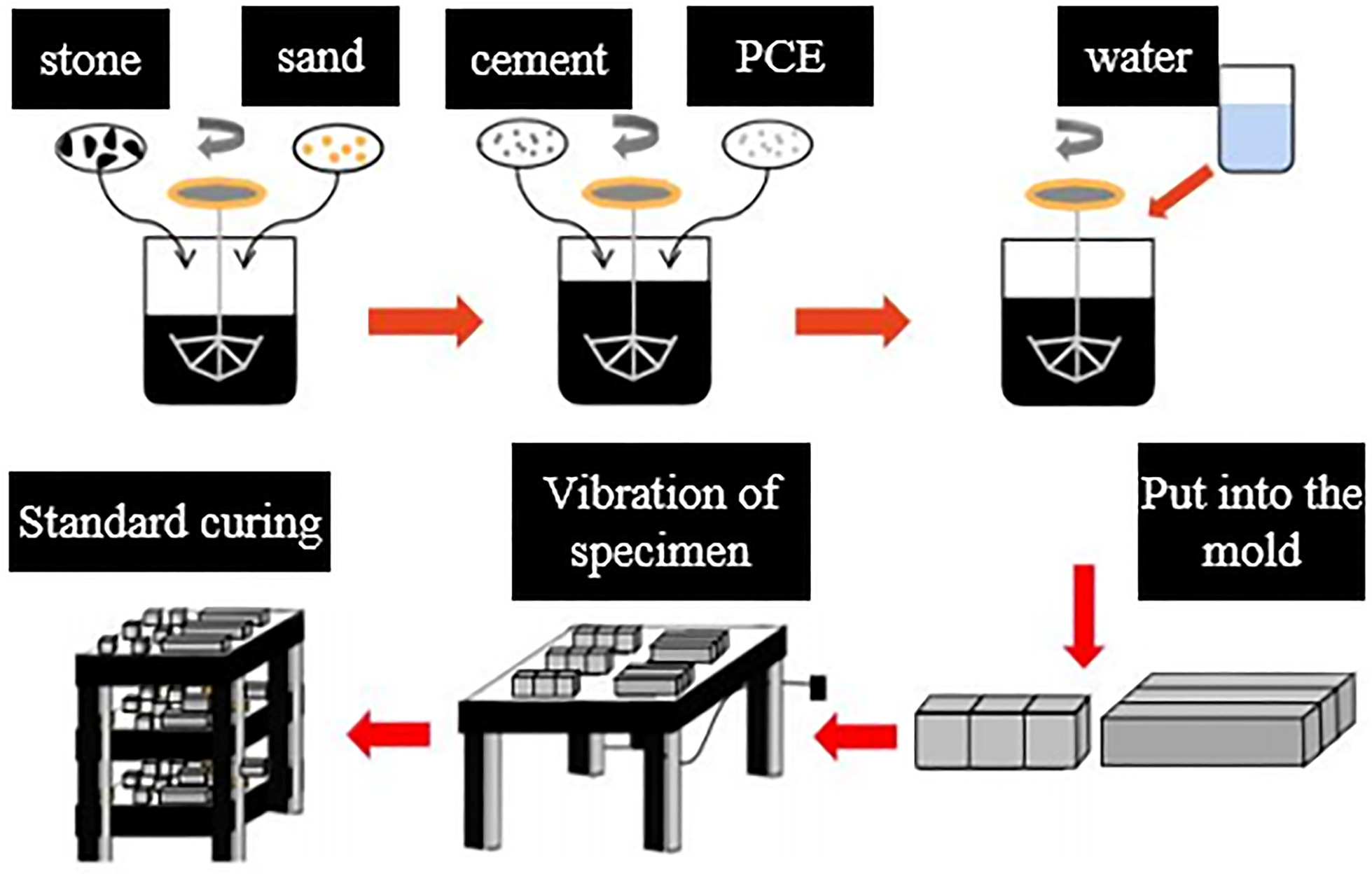
Experimental Arrangement
The single-factor tests consisted of four groups: waste landfill leachate full immersion (Group S), full immersion corrosion (Groups Y1 and Y2), and freeze–thaw cycle (Group D) (Table 2). The coupling tests consisted of three groups: waste landfill leachate coupled with freeze–thaw cycle groups (SD and DS) and compound salt solution coupled with freeze–thaw cycles (Group YD) (Table 3).
Group | Use for compression tests | Use for flexural tests | ||||||
---|---|---|---|---|---|---|---|---|
30 days | 60 days | 90 days | 120 days | 30 days | 60 days | 90 days | 120 days | |
Y1 | 156.6 | 161.8 | 160.9 | 160.3 | 634.6 | 631.3 | 632.7 | 632.2 |
158.7 | 160.4 | 160.2 | 158.7 | 635.8 | 635.4 | 633.2 | 635.6 | |
157.9 | 161.1 | 161.3 | 157.9 | 633.7 | 635.6 | 632.1 | 633.2 | |
Y2 | 158.4 | 159.3 | 161.4 | 159.0 | 634.7 | 634.1 | 632.1 | 632.5 |
158.4 | 158.4 | 159.5 | 158.1 | 636.1 | 636.3 | 636.3 | 631.5 | |
157.8 | 160.4 | 159.9 | 157.8 | 636.2 | 634.5 | 632.5 | 633.9 | |
S | 158.3 | 158.5 | 160.7 | 160.0 | 633.6 | 636.7 | 635.8 | 635.9 |
159.9 | 156.9 | 158.5 | 159.0 | 633.7 | 634.6 | 633.5 | 634.6 | |
157.0 | 157.3 | 158.9 | 159.7 | 634.1 | 634.9 | 633.8 | 632.7 | |
D | 159.2 | 158.4 | 159.1 | 158.9 | 635.1 | 634.7 | 634.9 | 633.8 |
158.6 | 159.3 | 159.6 | 160.1 | 635.9 | 364.5 | 633.8 | 636.2 | |
158.6 | 159.1 | 160.3 | 159.3 | 636.2 | 635.3 | 634.4 | 635.7 |
Note: The values given are mass values in grams.
Group | Use for compression tests | Use for flexural tests | ||||||
---|---|---|---|---|---|---|---|---|
I | II | III | IV | I | II | III | IV | |
SD | 158.7 | 158.8 | 159.9 | 160.3 | 635.6 | 634.5 | 636.4 | 639.0 |
159.6 | 159.4 | 159.4 | 160.8 | 636.8 | 633.3 | 635.1 | 639.2 | |
158.8 | 159.1 | 159.5 | 160.8 | 636.5 | 633.5 | 636.5 | 638.5 | |
DS | 158.2 | 159.1 | 160.3 | 159.7 | 635.8 | 636.3 | 634.2 | 636.5 |
158.4 | 158.6 | 157.9 | 160.3 | 634.9 | 634.9 | 636.2 | 638.3 | |
159.5 | 160.1 | 159.4 | 158.7 | 636.0 | 635.6 | 636.3 | 634.2 | |
YD | 158.4 | 158.5 | 158.2 | 160.2 | 638.4 | 637.1 | 637.5 | 638.0 |
157.6 | 159.4 | 158.7 | 160.0 | 637.5 | 636.0 | 637.7 | 635.2 | |
158.2 | 158.5 | 159.0 | 160.5 | 639.2 | 637.8 | 633.4 | 637.9 |
Note: The values given are mass values in grams.
Methods
Compression Test
Compression tests were conducted in accordance with Chinese standard GB/T 17671-1999 (GB 1999). The tests were performed using a liquid display full-automatic compression and flexure testing machine (YAW-Z300S Hengruijin Testing Machine Company, Jinan, Shandong, China) with a maximum compressive load of 300 KN. The compressive strength was obtained using the following equation:where fcc = compressive strength of concrete (MPa); F = load at failure (N); and A = area of the specimen under pressure (mm2). The arithmetic mean of the readings from the three specimens was the strength value for the group of specimens and was accurate to 0.1 MPa. Among the three measured values, if the difference between the maximum or minimum of any of the measured values and the median value is more than 15% of the median value, the median value will be considered as the measured value; when the difference between the maximum and minimum of the measured values and the median value are both greater than 15% of the median value, the test results of the group are invalid.
(1)
Flexural Test
The flexural tests were conducted in accordance with the Chinese standard GB/T 17671-1999 (GB 1999). Based on the test, a liquid display full-automatic compression and flexure testing machine (YAW-Z300S Hengruijin Testing Machine Company, Jinan, Shandong, China) with a maximal load of 50 kN was used. During the test, the load was applied in a continuous and uniform manner at a rate of 50 N/s. The load on the specimens was recorded in real time by the computer, using the same principles as for compressive strength, and was accurate to 0.1 MPa:where ff = flexural strength of the concrete (MPa); F = load at which the specimen breaks (N); l = span between supports (mm); and b = side length of the square section (mm).
(2)
Testing methods for landfill leachate, scanning electron microscopy (SEM) and X-ray diffraction (XRD) are described as follows:
1.
Ion chromatography and spectrophotometry were used to detect the concentration of substances in the landfill leachate. An appropriate amount of landfill leachate was taken, and the centrifuge speed was adjusted to 4,000 rpm for 3 min; the supernatant was diluted 1,000-fold.
2.
SEM was used to characterize the surface and internal structures of the concrete. A concrete sample with a volume of approximately 0.5 cm3 was taken, dried in a 50°C oven for 12 h, and immersed in anhydrous ethanol to stop hydration. A surface gold spray treatment was then performed under vacuum conditions.
3.
XRD was used to detect the phases in concrete at scanning angles ranging from 10° to 80°. A dry concrete sample was obtained, and the coarse aggregate was removed, ground, and sieved before being loaded into a press for detection.
Results and Discussion
Mechanical Properties of Concrete under Single-Factor Loading
This study involved a single-factor experiment that was divided into four groups: the Full immersion group S, which was soaked in the landfill leachate (with the addition of 5% sodium chloride and 5% sodium sulfate); the Full immersion group Y1, which was soaked in a 5% sodium chloride solution; the Full immersion group Y2, which was soaked in a 5% sodium sulfate solution; and the Freeze–thaw cycle group D, which underwent a daily cycle of freezing for 12 h and thawing for 12 h. The corrosion periods for each single-factor experimental group were 30, 60, 90, and 120 days. The results of the compressive strength test of the concrete after being soaked in different single-factor corrosion solutions are shown in Fig. 2, and the results of the flexural strength test are shown in Fig. 3.
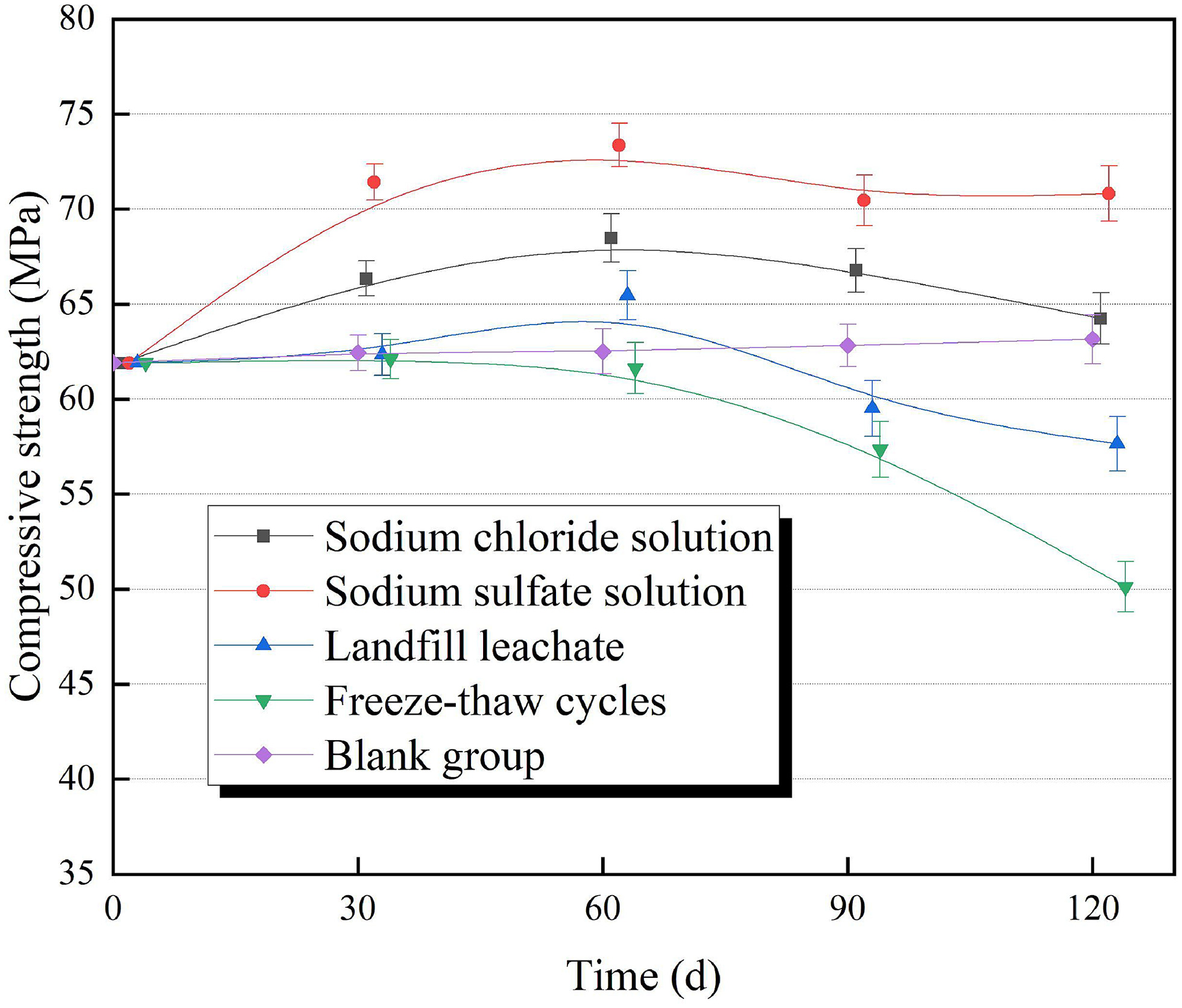
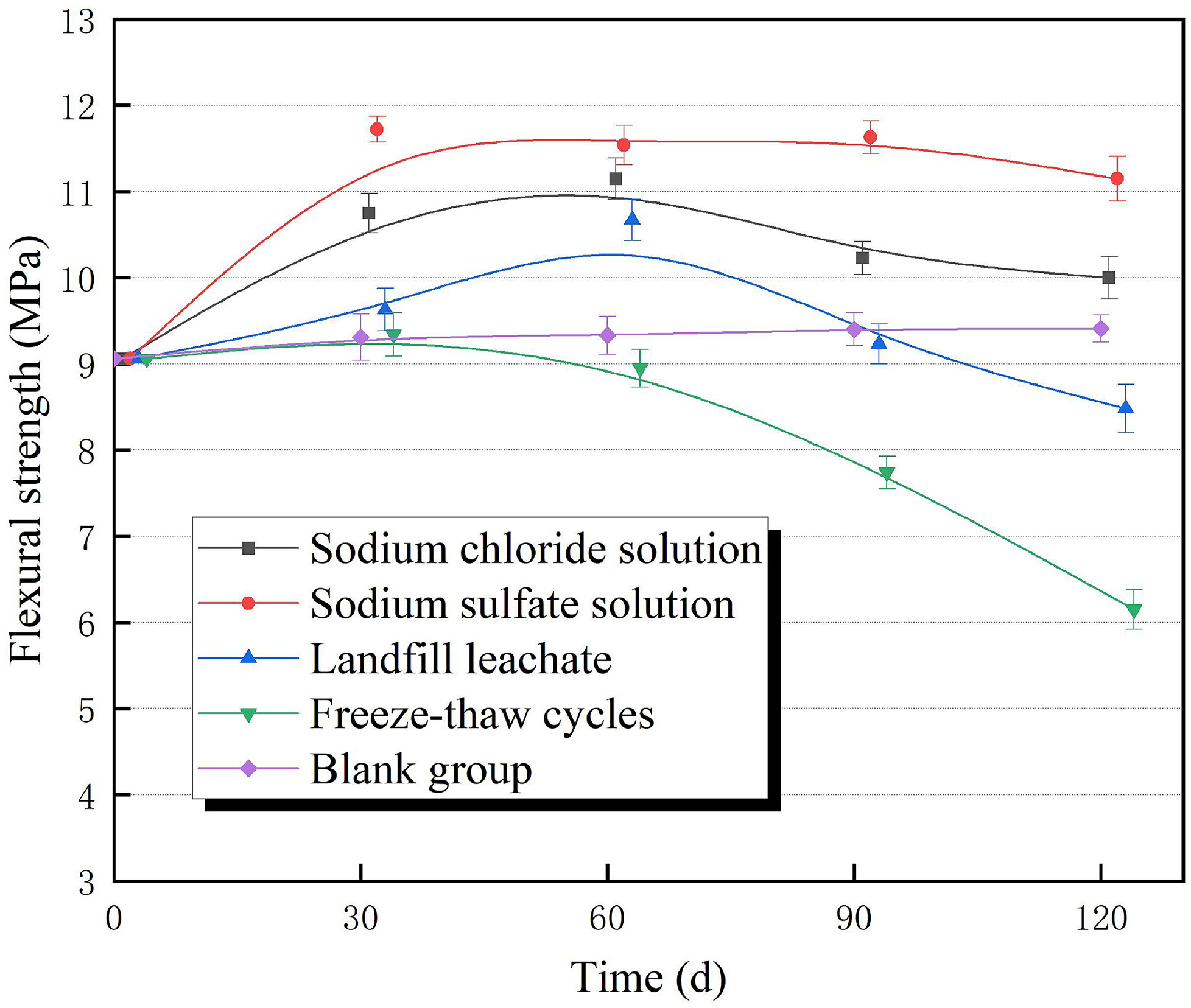
The compressive strength of the Freeze–thaw cycle test group D decreased in a single downward trend; after 120 freeze–thaw cycles, it decreased by 17.19%. The trend of the compressive strength variation in Test groups Y1, Y2, and S subjected to three different types of soaking corrosion was divided into two stages. The first stage showed an upward trend, with the smallest increase in compressive strength observed in the S group samples corroded by landfill leachate and the largest increase observed in the Y2 group samples corroded by the sodium sulfate solution. The compressive strengths of the three sample groups increased by 10.61%, 18.51%, and 5.73%, respectively. The second stage showed a downward trend, and the decreases in the compressive strengths of the S group samples were much greater than those of the Y1 and Y2 groups. After 120 days of corrosion, the compressive strengths of the S group samples decreased by 6.88%, whereas those of the Y1 and Y2 group samples increased by 3.78% and 14.39%, respectively. Therefore, the effect of landfill leachate on the compressive strength of concrete was greater than that of sodium sulfate and sodium chloride solutions, consistent with the conclusion drawn by Feng (2022).
The flexural strength of concrete under the influence of a single factor was studied, and the experimental results showed that the trend of flexural strength with age was similar to that of compressive strength; however, the magnitude of the variation was larger. In the first stage, the flexural strengths of the Y1, Y2, and S groups increased by 23.07%, 29.36%, and 17.77%, respectively. After 120 days of corrosion, the flexural strengths of the Y1 and Y2 groups increased by 10.37% and 23.73%, respectively, whereas those of the D and S groups decreased by 32.12% and 6.40%, respectively. Therefore, within a certain period of time, the corrosion of concrete by landfill leachate was less than that of freeze–thaw cycles; however, compared with the corrosion of concrete by the sodium sulfate and sodium chloride solutions, the corrosion of concrete by landfill leachate was greater. This may be because the salt ions contained in the landfill leachate reacted to generate calcium aluminate, which filled the pores and rendered the internal structure of the concrete dense, increasing the strength of the concrete in the early stages (Xia et al. 2023). With the extension of the corrosion age, other substances in the landfill leachate severely corroded the concrete, accelerating the decline in compressive and flexural strengths. We found that freeze–thaw cycles had the greatest impact on concrete, although landfill leachate and salt solutions also had significant impacts. Based on this phenomenon, we investigated the corrosion characteristics of concrete by conducting coupled tests to compare the effects of landfill leachate in cold regions.
Mechanical Properties of Concrete Subjected to Coupling Factors
Three sets of coupling tests were conducted, divided into SD and DS groups under the coupling of landfill leachate and freeze–thaw cycles, to simulate the different conditions of concrete corrosion and freeze–thaw cycles. The SD group was first immersed in landfill leachate and then subjected to freeze–thaw cycles, while the DS group was the opposite. The YD group was coupled with a composite salt solution (5% sodium chloride + 5% sodium sulfate solution) and subjected to freeze–thaw cycles. Each group was evaluated for four cycles, with one period consisting of 30 days of soaking and 25 freeze–thaw cycles. The compressive strength test results of concrete after the coupling factor action are shown in Fig. 4, and the flexural strength test results are shown in Fig. 5.
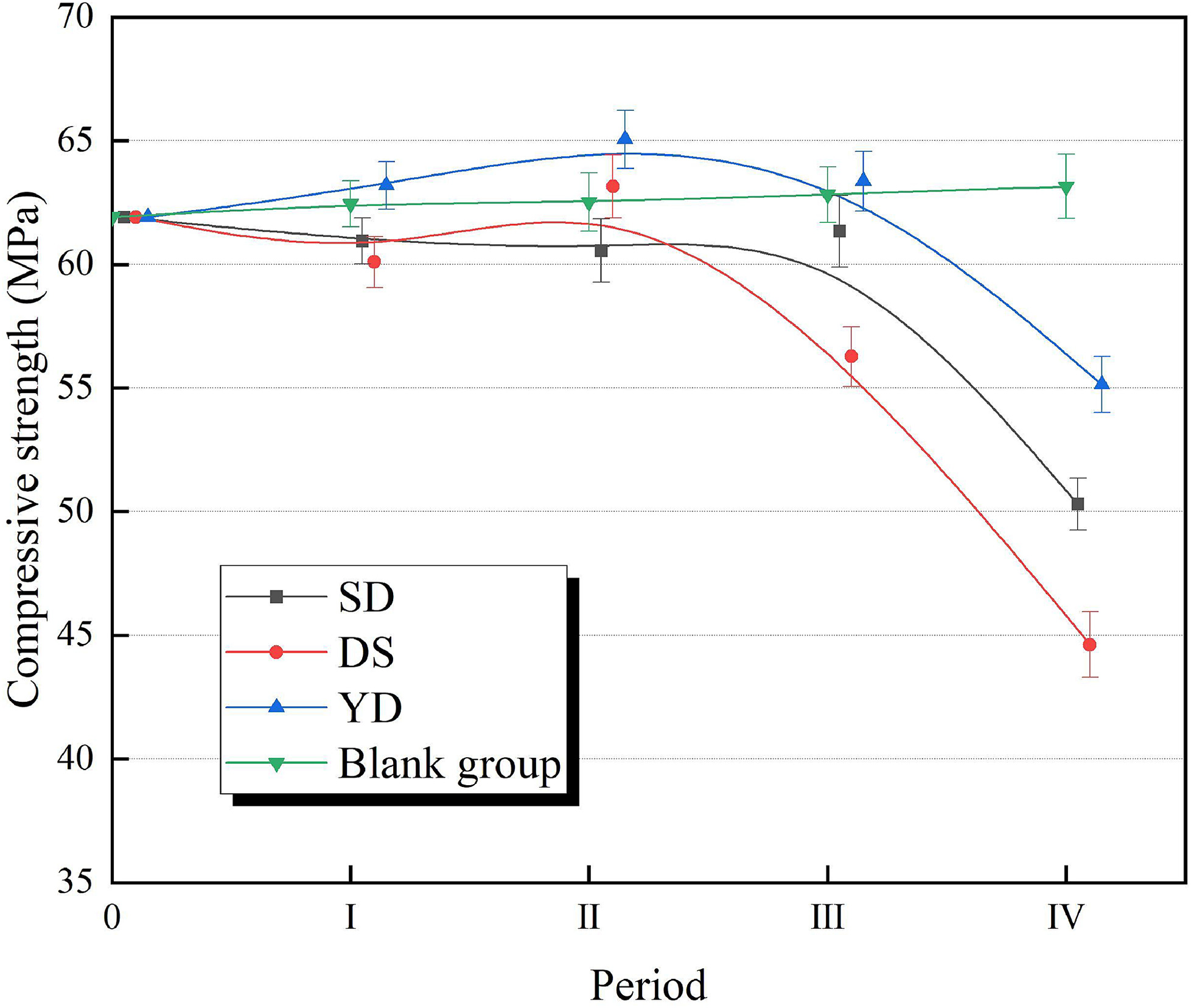
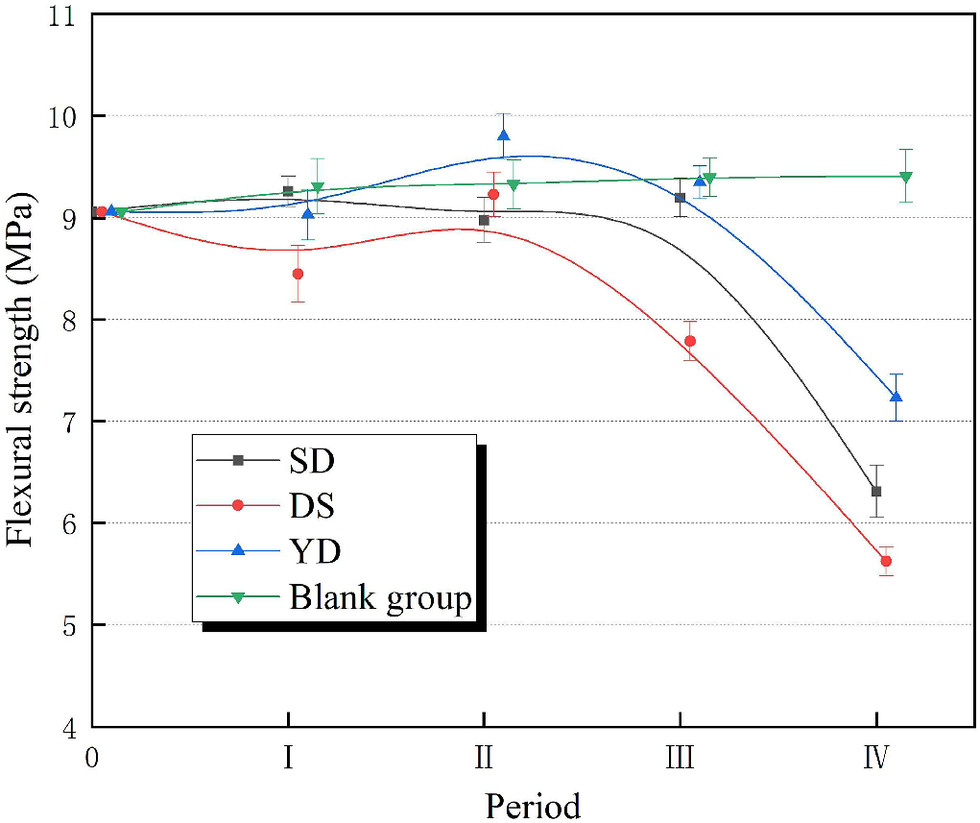
The compressive strength results of the coupling test groups showed that in the first cycle, the compressive strength of group YD was the highest, and the compressive strength of group DS was lower than that of group SD. In the second cycle, the compressive strengths of the DS and YD groups reached their peaks, increasing by 2.00% and 5.09%, respectively, whereas that of the SD group showed a slow downward trend, decreasing by 2.19%. In the third cycle, the compressive strength of group SD reached its peak, whereas those of groups DS and YD decreased significantly. After four corrosion cycles, the compressive strengths of the three coupled test groups, SD, DS, and YD, decreased significantly. Among them, the freeze–thaw cycle and landfill leachate coupling test group DS was the most affected, with a reduction of 18.74%, and the freeze–thaw cycle and salt solution coupling test group YD was the least affected, with a reduction of 10.94%. Therefore, concrete subjected to freeze–thaw cycles and then corroded by landfill leachate was the most severely damaged.
The results of the flexural strength tests for the coupled test groups showed that the flexural strengths of the DS and YD groups reached peak values in the second cycle, increasing by 1.88% and 8.17%, respectively. The flexural strength of the SD group reached its peak value in the third cycle, increasing by 1.55%. In the fourth cycle, the flexural strengths of the three coupled test groups, SD, DS, and YD, decreased significantly, with reductions of 30.35%, 37.97%, and 26.7%, respectively. This may be attributable to the fact that the freeze–thaw cycle first damaged the concrete structure, making it easier for inorganic salt ions to enter the specimen and react with the concrete matrix to generate calcium aluminate and other hydration products with expansive properties, filling the cracks in the specimen and continuously increasing the compressive and flexural strengths of the concrete until they reached their peak values (Xiao et al. 2019). As the freeze–thaw cycle and immersion corrosion continued, the expansive materials and frozen water pressure generated expansion stress inside the concrete, exacerbating the damage inside the concrete and easily allowing corrosion solutions to enter the concrete, creating a cycle that ultimately leads to a rapid decrease in concrete strength (Jiang et al. 2015).
Based on the aforementioned experimental results, the damage to concrete caused by the coupling effect of landfill leachate and freeze–thaw cycles was determined to be more severe than that caused by the coupling effect of the salt solution and freeze–thaw cycles. Additionally, the concrete that was first subjected to freeze–thaw cycles was more affected than that subjected to landfill leachate corrosion, indicating that microorganisms and other acidic substances in the landfill leachate, in addition to inorganic salt ions, could also damage the internal structure and significantly impact the strength of concrete. Furthermore, compared with concrete that was first corroded by landfill leachate, concrete that was first subjected to freeze–thaw cycles reached its peak and entered a damaged state earlier.
Apparent and Microscopic Changes in Concrete after Corrosion
In the coupling test group, the apparent damage characteristics of the concrete specimens under different corrosion conditions and action cycles are shown in Fig. 6.
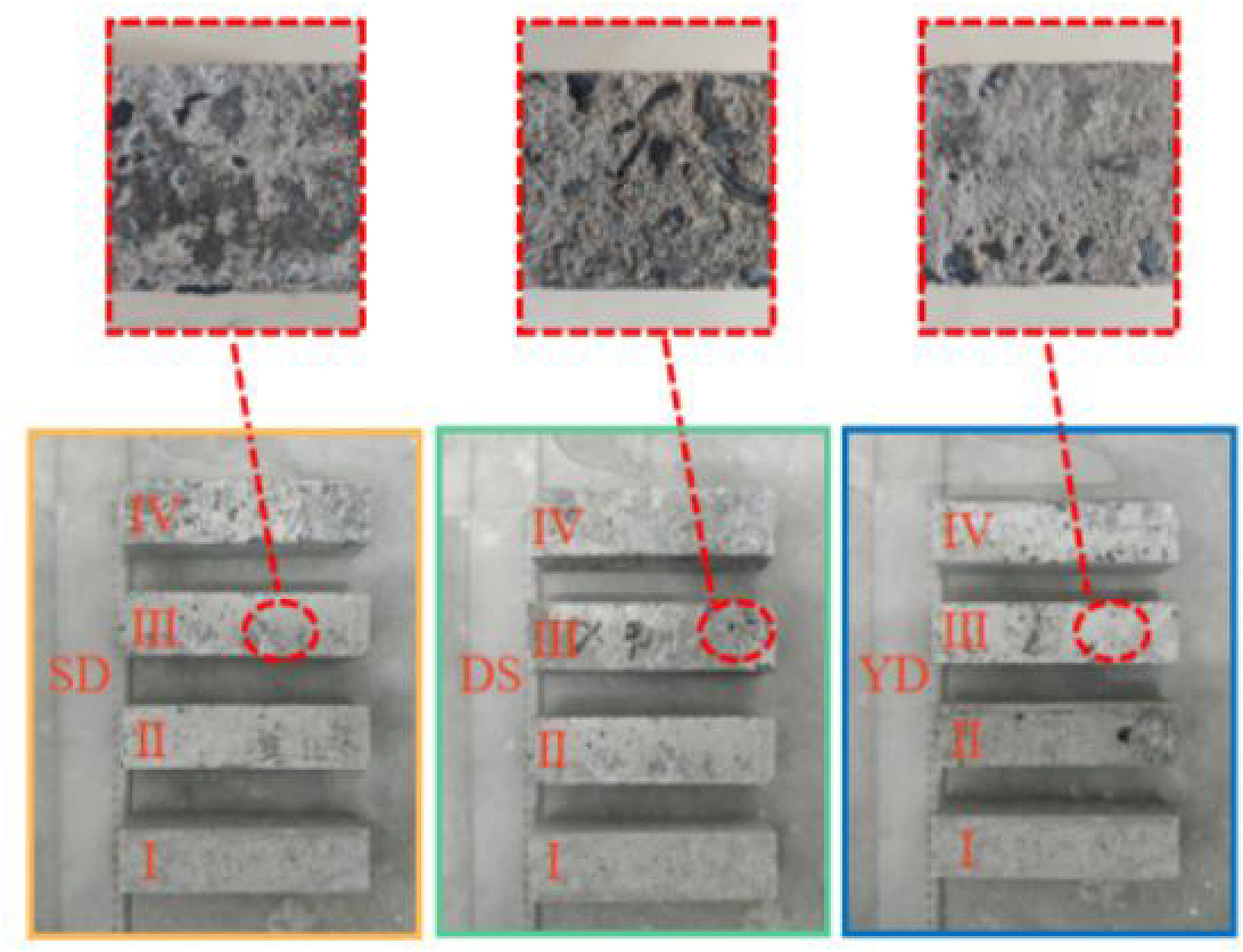
As the action cycle progressed, the surface of the specimen became rough, and the peeling on the cement mortar protective layer on the surface became increasingly severe. This is because the stable structure of the alkaline cementitious material on the specimen surface was destroyed, resulting in a loss of its ability to protect the aggregates. After the third cycle, all three test groups exhibited intensified cement mortar peeling and exposure to coarse aggregates. The calculated mass loss rates showed that the DS test group had the most severe concrete specimen damage, with a mass loss rate of 0.85%, followed by the SD test group, with a mass loss rate of 0.73%, whereas the YD test group had the least damage, with a mass loss rate of 0.55%.
Based on the results of the mechanical tests, significant differences were observed between the first and third cycles. To further investigate the corrosion mechanism, concrete samples under different corrosion environments were analyzed using SEM (Fig. 7). The internal structure of Sample group S30 was dense and contained only a small number of micropores, whereas Sample group S90 produced needle-shaped and debris-like products with slight cracks. The blank group of concrete contained a large amount of C–S–H gel and plate-like Ca(OH)2 crystals, and its structure was dense with no significant cracks. In the coupling test of the first cycle, Sample groups SDI and DSI had numerous plate- and fiber-like substances distributed internally, accompanied by slight cracks and a small amount of needle-like substances, resulting in slight damage to the concrete compared with Sample group S30. In the coupling test of the third cycle, compared with Sample groups SDI and DSI, both Sample groups SDIII and DSIII produced more new cracks and pores inside the concrete, filled with a large number of needle-shaped and debris-like products with higher porosity. Sample group DSIII had larger cracks and more debris-like products with a more severe level of damage than Sample group SDIII. Combined with the results of the macroscopic mechanical tests, concrete subjected to freeze–thaw cycles first suffered more severe damage than concrete subjected to landfill leachate corrosion; upon comparing Figs. 7(d and h), we found that numerous needle-shaped ettringite and debris-like gypsum were inside the concrete of the YDIII test group, with obvious pores and cracks. In contrast, the pores and cracks in the SDIII test group were much more numerous than those in the YDIII test group and were filled with many needle-shaped and debris-like products. Based on these phenomena, the coupling effect of freeze–thaw cycles and landfill leachate from waste landfill is greater than the coupling effect of freeze–thaw cycles and salt solution on concrete. In the early stages of the experiment, inorganic salt ions in the landfill leachate gradually penetrated the concrete specimens, forming crystalline substances that filled the pores of the concrete specimens and made the internal structure of the concrete denser. As the test cycle progressed, the external cement mortar began to peel off and an increasing number of water molecules and landfill leachate entered the specimens. The inorganic salt ions in the landfill leachate consumed the hydration products in the concrete specimens, such as C–S–H and calcium hydroxide, to generate expansible needle-shaped ettringite and gypsum. This, in turn, caused more micropores and cracks under the influence of freeze–thaw cycles (Zhao et al. 2016). As the test cycles increased, the degradation rates of the concrete specimens increased. These phenomena are consistent with the existing conclusions that inorganic salt ions fill cracks and pores in concrete during the early and middle stages of freeze–thaw cycles, and in the later stages, they have a significant mutual promotional effect on the damage caused by freeze–thaw cycles to concrete (Luo et al. 2018).
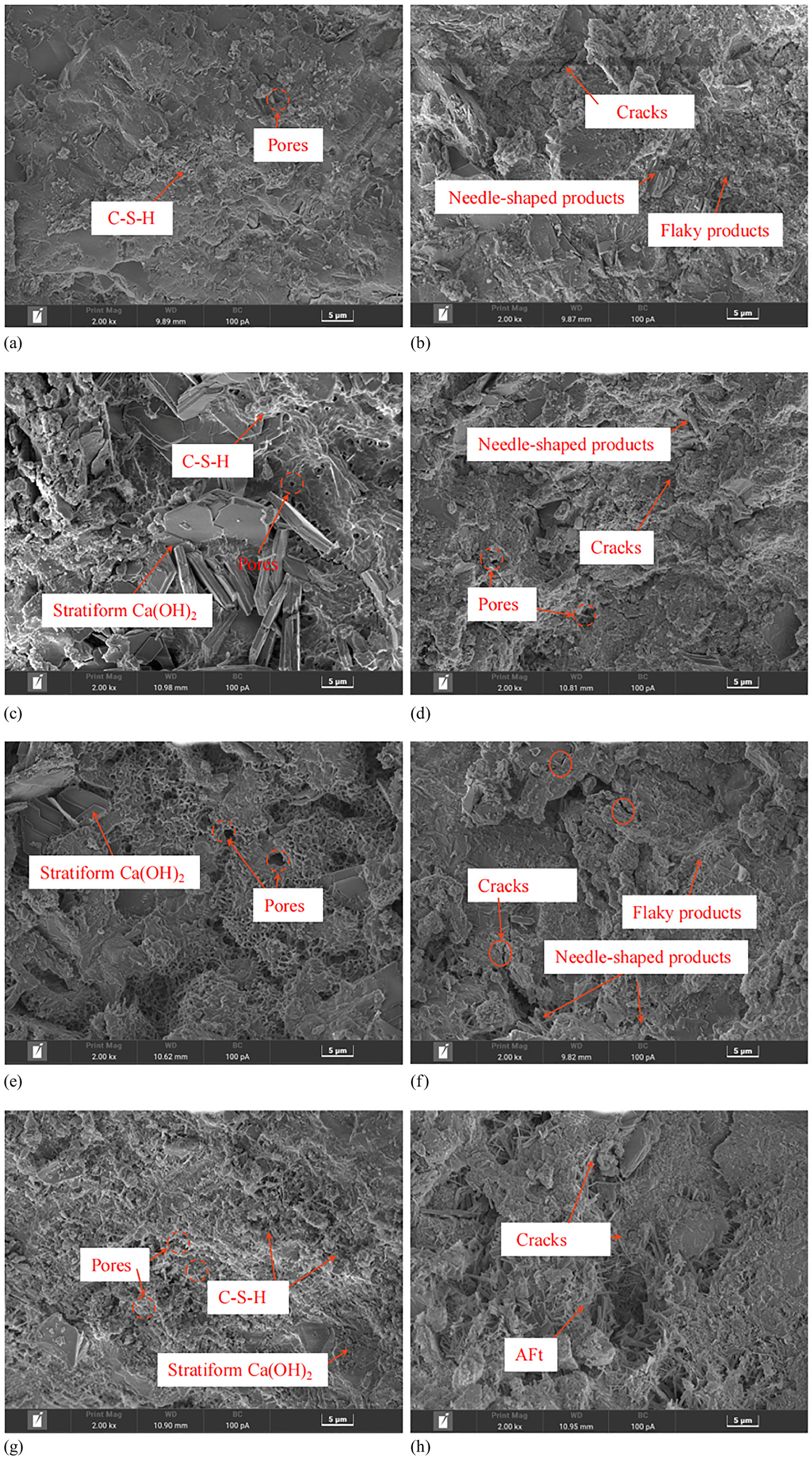
The XRD phase analysis diagram in Fig. 8 shows that the concrete corroded by landfill leachate contained not only C–S–H but also ettringite at a corrosion age of 30 days. At this time, the ettringite filled the pores and cracks inside the concrete specimens, improving its internal structure and making them denser. Concrete specimens corroded by landfill leachate and those corroded by salt in the third period in severely cold regions both contained ettringite and gypsum, which verifies the previous speculation, as shown in Fig. 9. As the corrosion age increased, the calcium hydroxide content inside the concrete specimens decreased continuously, and more needle-shaped ettringite and fragmentary gypsum appeared, generating outward expansive stress. When the expansive stress exceeded the tensile stress inside the concrete, numerous microcracks occurred, damaging its internal structure (Liu et al. 2020). Compared with single landfill leachate corrosion, the concrete specimens in the coupling test group had more cracks within owing to the freeze–thaw cycles, which increased the contact area between the corrosion solution and concrete and accelerated the generation of ettringite and gypsum. The combined action of freeze–thaw stress and expansive stress ultimately led to concrete damage, which explains the accelerated decline of the concrete's macroscopic mechanical properties in the later stage from a microscopic perspective.
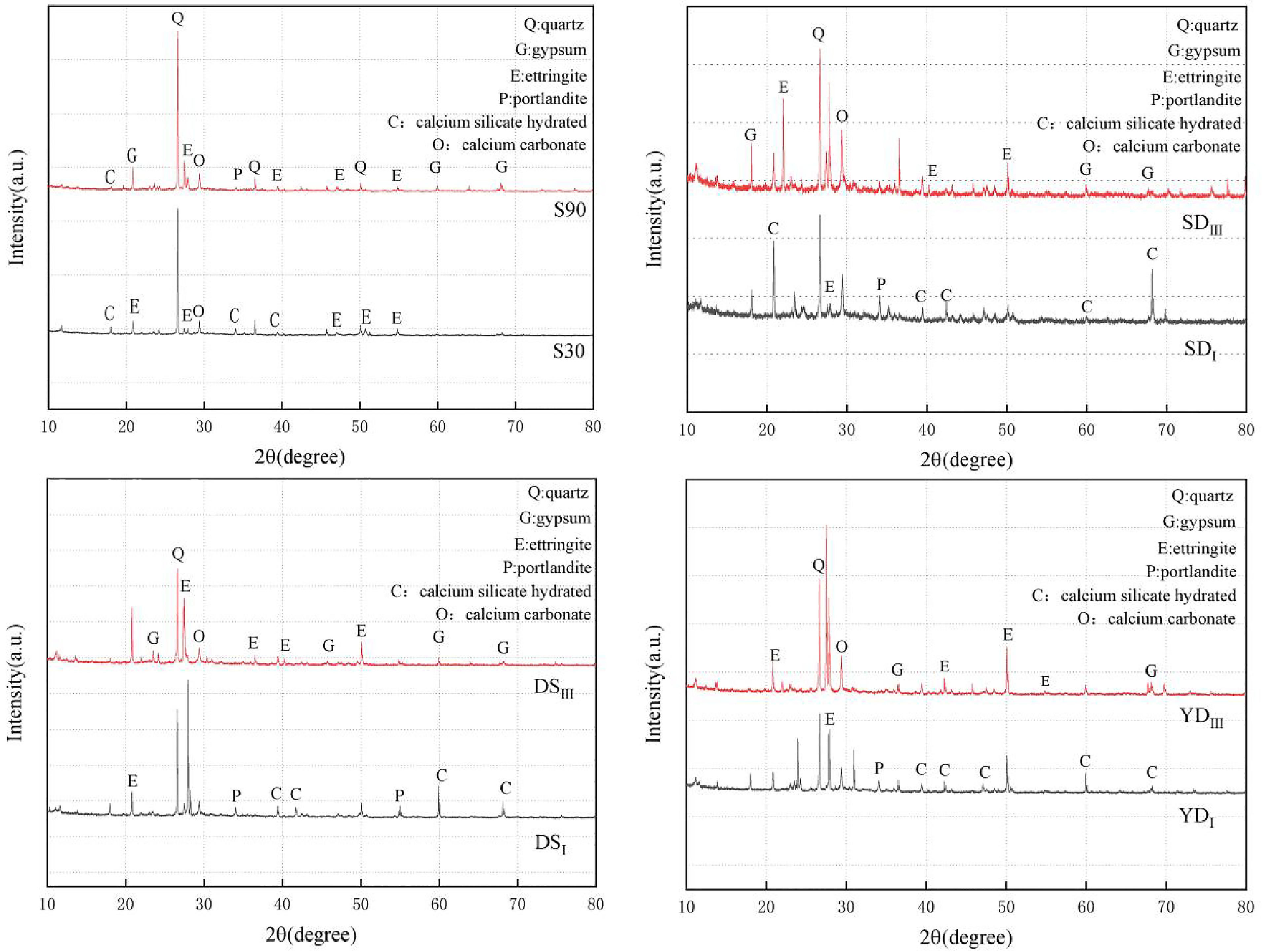
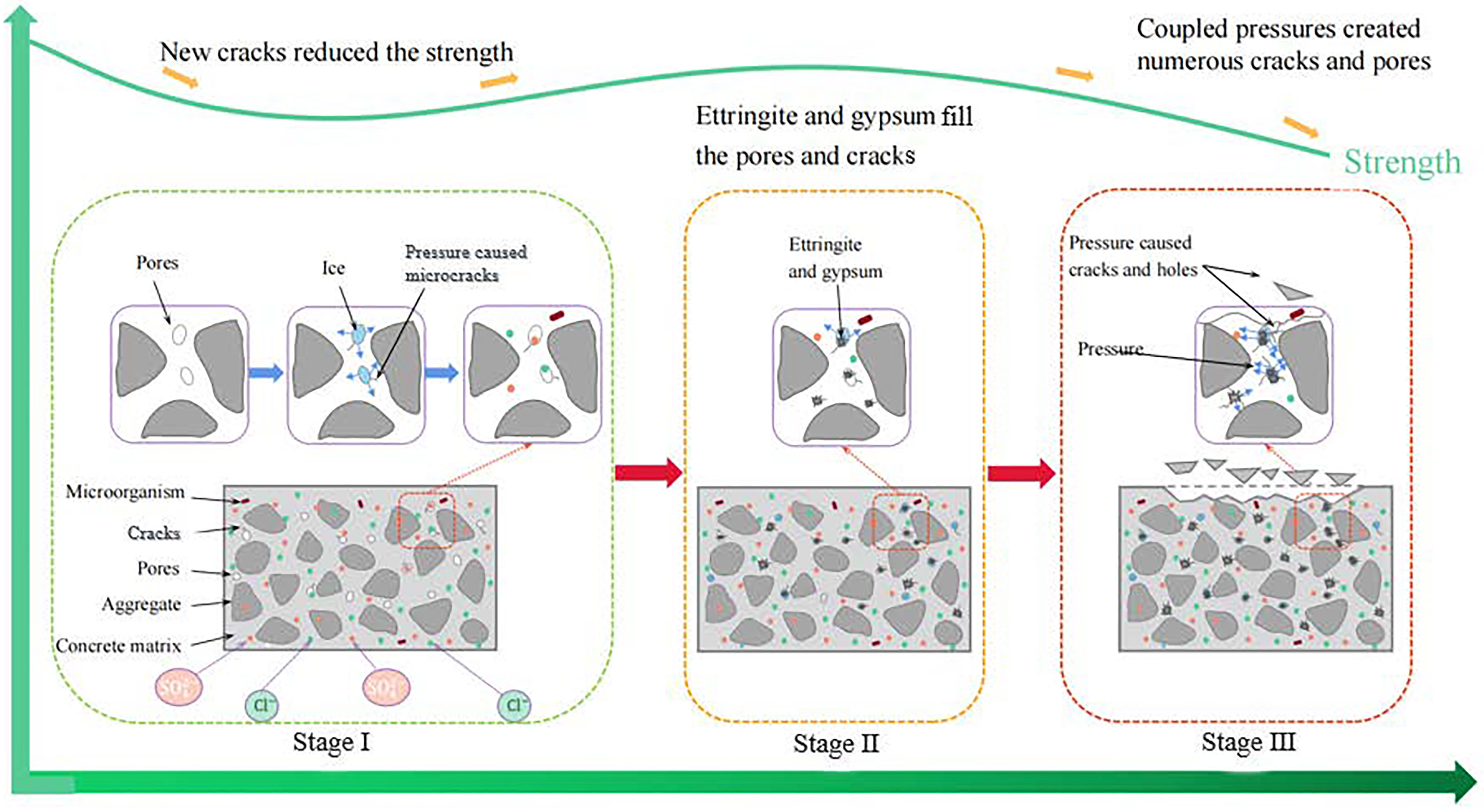
Conclusions
This study establishes single-factor and coupled-factor experimental groups to analyze the corrosion mechanisms of concrete by landfill leachate in cold regions based on the detection of the mechanical properties of concrete, combined with its apparent morphological and microstructural characteristics. The main conclusions are as follows:
1.
Single-factor experiments showed that under continuous corrosion by landfill leachate, the compressive and flexural strengths of concrete specimens were divided into two stages: a linear increase stage and a slow decrease stage. However, the strength of the specimens corroded by landfill leachate throughout the entire process was always lower than that of the specimens corroded by the sodium chloride and sodium sulfate solutions. Under the action of freeze–thaw cycles, the strength of the specimens decreased slowly in the early stage and rapidly in the later stage, and the degree of influence on the mechanical properties of the concrete was higher than that of the other three experimental groups.
2.
Coupled experiments showed that concrete specimens subjected to freeze–thaw cycles before being corroded by landfill leachate had the greatest loss in strength. Compared with the single corrosion of landfill leachate and the single freeze–thaw cycle, the compressive and flexural strengths of the specimens decreased significantly, and the flexural strength decreased more than the compressive strength. Therefore, for concrete structures that could be subjected to the coupled corrosion of freeze–thaw cycles and landfill leachate, avoiding large bending stresses is necessary. Protection methods that improve the flexural strength of concrete should be prioritized.
3.
The coupling effect of freeze–thaw cycles and landfill leachate can exacerbate the peeling of cement mortar on the surface of concrete specimens and the exposure of coarse aggregates. Compared with composite salt solutions, inorganic salt ions, microorganisms, and other acidic substances in landfill leachate can consume hydration products in concrete specimens, causing more severe corrosion. Inorganic salt ions react to produce expansive needle-like ettringite and granular gypsum. Under the action of freeze–thaw cycles, more micropores and cracks are generated, causing severe damage to the concrete. Therefore, freeze–thaw cycles in cold regions significantly exacerbate the corrosion of concrete by landfill leachate.
Data Availability Statement
All data, models, and codes generated or used during the study appear in the published article.
Acknowledgments
This research was supported by the Heilongjiang Academy of Sciences (YZQY2023GJS01), Heilongjiang Province Basic Scientific Research (KJCXYB201703 and KJCXYB201704), and the National Natural Science Foundation of China (Grant No. 51708521). Tiefu Xu and Shuaishuai Bi contributed equally to this work.
References
Amor, C., E. D. Torres-Socías, J. A. Peres, M. I. Maldonado, I. Oller, S. Malato, and M. S. Lucas. 2015. “Mature landfill leachate treatment by coagulation/flocculation combined with Fenton and solar photo-Fenton processes.” J. Hazard. Mater. 286: 261–268. https://doi.org/10.1016/j.jhazmat.2014.12.036.
Anand, N., and S. G. Palani. 2022. “A comprehensive investigation of toxicity and pollution potential of municipal solid waste landfill leachate.” Sci. Total Environ. 838: 155891. https://doi.org/10.1016/j.scitotenv.2022.155891.
Chen, D., Y. Deng, J. Shen, G. Sun, and J. Shi. 2021. “Study on damage rules on concrete under corrosion of freeze‒thaw and saline solution.” Constr. Build. Mater. 304: 124617. https://doi.org/10.1016/j.conbuildmat.2021.124617.
Chen, H., Y. Cao, Y. Liu, Y. Qin, and L. Xia. 2023. “Enhancing the durability of concrete in severely cold regions: Mix proportion optimization based on machine learning.” Constr. Build. Mater. 371: 130644. https://doi.org/10.1016/j.conbuildmat.2023.130644.
Cheng, L., L. Shi, L. Yi, and W. Zhao. 2022. “Study on mechanical properties and constitutive relationship of concrete corroded by hydrochloric acid under cyclic load.” Sustainability 14: 14692. https://doi.org/10.3390/su142214692.
Diao, B., Y. Sun, S. Cheng, and Y. Ye. 2011. “Effects of mixed corrosion, freeze‒thaw cycles, and persistent loads on behavior of reinforced concrete beams.” J. Cold Reg. Eng. 25: 37. https://doi.org/10.1061/(ASCE)CR.1943-5495.0000019.
Dubravka, B., S. Marijana, and C. Igor. 2010. “Corrosion of concrete by microorganisms.” J. Chin. Ceram. Soc. 38: 1741–1745. https://doi.org/10.14062/j.issn.0454-5648.2010.09.031.
Feng, W. 2022. “Evaluation of macro- and meso-mechanical properties of concrete under the aggressiveness of landfill leachate.” Sci. Rep. 12: 3976. https://doi.org/10.1038/s41598-022-07418-7.
GB (Guobiao Standards). 1999. Method of testing cements—Determination of strength, the state bureau of quality and technical supervision. GB/T 17671. Beijing: GB.
Gong, L., X. Yu, Y. Liang, X. Gong, and Q. Du. 2023. “Multi-scale deterioration and microstructure of polypropylene fiber concrete by salt freezing.” Case Stud. Constr. Mater. 18: e01762. https://doi.org/10.1016/j.cscm.2022.e01762.
Jiang, L., D. Niu, L. Yuan, and Q. Fei. 2015. “Durability of concrete under sulfate attack exposed to freeze‒thaw cycles.” Cold Reg. Sci. Technol. 112: 112–117. https://doi.org/10.1016/j.coldregions.2014.12.006.
Li, B., J. Mao, T. Nawa, and Z. Liu. 2016. “Mesoscopic chloride ion diffusion model of marine concrete subjected to freeze‒thaw cycles.” Constr. Build. Mater. 125: 337–351. https://doi.org/10.1016/j.conbuildmat.2016.08.052.
Li, L. G., J. Y. Zheng, P. L. Ng, J. Zhu, and A. K. H. Kwan. 2019. “Cementing efficiencies and synergistic roles of silica fume and nano-silica in sulphate and chloride resistance of concrete.” Constr. Build. Mater. 223: 965–975. https://doi.org/10.1016/j.conbuildmat.2019.07.241.
Li, T., and H.-H. Huang. 2021. “Probabilistic quantitative analysis on the contents of sulfate corrosion products in concrete.” Constr. Build. Mater. 275: 122134. https://doi.org/10.1016/j.conbuildmat.2020.122134.
Lines, S. J., D. A. Rothstein, B. Rollins, and C. Alt. 2021. “Microbially induced corrosion of concrete.” Concr. Int. 43: 28–32.
Liu, C., J. Wang, J. Cai, and Y. Rong. 2009. “Analysis of the case of landfill cover system damage.” China Water Wastewater 25: 18–22. https://doi.org/10.3321/j.issn:1000-4602.2009.24.005.
Liu, P., Y. Chen, W. Wang, and Z. Yu. 2020. “Effect of physical and chemical sulfate attack on performance degradation of concrete under different conditions.” Chem. Phys. Lett. 745: 137254. https://doi.org/10.1016/j.cplett.2020.137254.
Luo, Q., D. X. Liu, P. Qiao, Q. G. Feng, and L. Z. Sun. 2018. “Microstructural damage characterization of concrete under freeze‒thaw action.” Int. J. Damage Mech. 27: 1551–1568. https://doi.org/10.1177/1056789517736573.
Miller, D. E., and S. M. Emge. 1997. “Enhancing landfill leachate recirculation system performance.” Pract. Period. Hazard. Toxic Radioact. Waste Manage. 1: 113–119. https://doi.org/10.1061/(ASCE)1090-025X(1997)1:3(113).
Nica, D., J. L. Davis, L. Kirby, G. Zuo, and D. J. Roberts. 2000. “Isolation and characterization of microorganisms involved in the biodeterioration of concrete in sewers.” Int. Biodeterior. Biodegrad. 46: 61–68. https://doi.org/10.1016/S0964-8305(00)00064-0.
Osman, K. M., F. M. Taher, A. Abd EL-Tawab, and A. S. Faried. 2021. “Role of different microorganisms on the mechanical characteristics, self-healing efficiency, and corrosion protection of concrete under different curing conditions.” J. Build. Eng. 41: 102414. https://doi.org/10.1016/j.jobe.2021.102414.
Park, J. Y., J. Lee, M. Lim, G.-M. Go, H.-B. Cho, H.-S. Lee, and Y.-H. Choa. 2021. “Structure-modulated CaFe-LDHs with superior simultaneous removal of deleterious anions and corrosion protection of steel rebar.” RSC Adv. 11: 10951–10961. https://doi.org/10.1039/D1RA00300C.
Ren, Z., D. Niu, J. Wu, J. Zhang, and N. Meng. 2018. “Effect of freezing‒thawing cycle on the durability of concrete under extreme environment conditions.” J. Xi’an Univ. Archit. Technol. 50: 220–224. https://doi.org/10.15986/j.1006-7930.2018.02.011.
Rustamov, S., S. Woo Kim, M. Kwon, and J. Kim. 2021. “Mechanical behavior of fiber-reinforced lightweight concrete subjected to repeated freezing and thawing.” Constr. Build. Mater. 273: 121710. https://doi.org/10.1016/j.conbuildmat.2020.121710.
Thornton, R. J., and F. C. Blanc. 1973. “Leachate treatment by coagulation and precipitation.” J. Environ. Eng. Div. 99: 535–544. https://doi.org/10.1061/JEEGAV.0000074.
Wan, M., and H. Wan. 2011. Influence of leachate contaminants on setting and hardening of concrete. Perlis, Malaysia: Univ. Malaysia Perlis.
Wang, C., H. Lu, D. Li, and J. Li. 2020. “Experimental study on the permeability and microstructure of remoulded silty clay corroded by landfill leachate.” Nat. Environ. Pollut. Technol. 19: 1241–1248. https://doi.org/10.46488/NEPT.2020.v19i03.039.
Wdowczyk, A., and A. Szymańska-Pulikowska. 2021. “Analysis of the possibility of conducting a comprehensive assessment of landfill leachate contamination using physicochemical indicators and toxicity test.” Ecotoxicol. Environ. Saf. 221: 112434. https://doi.org/10.1016/j.ecoenv.2021.112434.
Wu, Q., Q. Ma, and X. Huang. 2021. “Mechanical properties and damage evolution of concrete materials considering sulfate attack.” Materials 14: 2343. https://doi.org/10.3390/ma14092343.
Xia, D., S. Yu, J. Yu, C. Feng, B. Li, Z. Zheng, and H. Wu. 2023. “Damage characteristics of hybrid fiber reinforced concrete under the freeze‒thaw cycles and compound-salt attack.” Case Stud. Constr. Mater. 18: e01814. https://doi.org/10.1016/j.cscm.2022.e01814.
Xiao, Q. 2010. Study on durability of concrete structure subjected to freezing‒thawing environment. Shaanxi, China: Xi'an Univ. of Architecture and Technology.
Xiao, Q. H., Z. Y. Cao, X. Guan, Q. Li, and X. L. Liu. 2019. “Damage to recycled concrete with different aggregate substitution rates from the coupled action of freeze‒thaw cycles and sulfate attack.” Constr. Build. Mater. 221: 74–83. https://doi.org/10.1016/j.conbuildmat.2019.06.060.
Yang, M., X. Luo, X. Yu, and H. Yao. 2005. “Study on foundation and safety of large building in Jinkou landfill site.” Chin. J. Rock Mech. Eng. 4: 628–637. https://doi.org/10.3321/j.issn:1000-6915.2005.04.014.
Zhang, R., L. Jin, M. Liu, X.-l. Du, and Y. Li. 2017. “Numerical investigation of chloride diffusivity in cracked concrete.” Mag. Concr. Res. 69: 850–864. https://doi.org/10.1680/jmacr.16.00511.
Zhao, L., J. Liu, W. Zhou, and H. Ji. 2016. “Damage evolution and mechanism of concrete erosion at sulfate environment in underground mine.” J. China Coal Soc. 41: 1422–1428. https://doi.org/10.13225/j.cnki.jccs.2015.1311.
Zhou, J., S. Yin, Q. Fu, Q. Wang, Q. Huang, and J. Wang. 2021. “Microbial-induced concrete corrosion under high-salt conditions: Microbial community composition and environmental multivariate association analysis.” Int. Biodeterior. Biodegrad. 164: 105287. https://doi.org/10.1016/j.ibiod.2021.105287.
Information & Authors
Information
Published In
Copyright
This work is made available under the terms of the Creative Commons Attribution 4.0 International license, https://creativecommons.org/licenses/by/4.0/.
History
Received: Jul 26, 2023
Accepted: Feb 26, 2024
Published online: Jul 16, 2024
Published in print: Dec 1, 2024
Discussion open until: Dec 16, 2024
ASCE Technical Topics:
- Compressive strength
- Concrete
- Corrosion
- Coupling
- Deterioration
- Engineering materials (by type)
- Engineering mechanics
- Environmental engineering
- Landfills
- Leachates
- Material mechanics
- Material properties
- Materials characterization
- Materials engineering
- Strength of materials
- Structural engineering
- Structural members
- Structural systems
- Waste management
- Waste sites
- Waste treatment
Authors
Metrics & Citations
Metrics
Citations
Download citation
If you have the appropriate software installed, you can download article citation data to the citation manager of your choice. Simply select your manager software from the list below and click Download.