Chapter 2
Vision for Tornado Design
Publication: State of the Art and Research Needs in Design for Tornadoes to Improve Community Resilience: Structural Engineering Institute Workshop Report
2.1 Current State of the Art
Under the assumption that the current design provisions contained in ASCE/SEI 7-22 reflect the state of the art, each breakout group assessed how those current provisions reflect their topic.
2.1.1 Tornado Climatology and Near-Surface Wind Characteristics
This breakout session examined engineering-relevant properties of the tornado hazard and the information needed to improve future editions of ASCE/SEI 7 and do fundamental research. In general, this work focused on two overarching objectives relevant to the meteorology community including
1.
Improvement of the characterization of tornadoes including their frequency, spatial and temporal variability, intensity, path characteristics, etc. (i.e., tornado climatology); and
2.
Improved understanding of the structure and evolution of the three-dimensional wind field in tornado vortices with a wide range of characteristics (i.e., near-surface wind characteristics).
ASCE/SEI 7-22 introduced design provisions for tornado loads. The current state of the art of tornado climatology and near-surface wind characteristics necessarily requires an interface between the meteorological and engineering communities.
Over the past decade, understanding of the near-surface wind field in tornadoes has improved dramatically in part due to field experiments (both during and after the event) and through numerical and experimental modeling. Datasets of high spatial and temporal resolution in situ and remote sensing (e.g., radar) of tornadoes have increased dramatically, allowing for even better understanding of such phenomena (e.g., Bodine and Griffin 2023). Field campaigns aimed at observing various tornado properties via ground-based and airborne observations have successfully expanded the available near-surface observations in tornadoes. In several cases, observations of the lowest 100 ft (30 m) of the tornado vortex have also been successfully collected (e.g., Wurman et al. 2013, McKeown et al. 2020), several of which were used in the development of the tornado wind profile for ASCE/SEI 7-22.
In addition to those observations of tornadoes, increases in the availability and efficiency of computational resources have produced numerical simulations of tornado-like vortices with very high spatial and temporal resolution. Novel numerical techniques including tornado simulations with quasi-realistic depictions of vortex turbulence (Bryan et al. 2017, Nolan et al. 2017), generated debris and debris loading (Bodine et al. 2016), and even simulations introducing interactions of the modeled vortices with surface obstacles including terrain and civil infrastructure (e.g., Satrio et al. 2020) have helped provide a more comprehensive understanding of realistic vortex behaviors particularly in situations where observations are not feasible (i.e., are too dangerous or difficult to collect).
From a tornado climatology perspective, improvements in remote sensing technologies have been the primary research driver related to improvements in post-tornado observations, as damage remains the source for an overwhelming majority of parameter estimates for tornado climatology. Research related to tornadoes spawned by quasi-linear convective systems (QLCS) has made major strides in terms of the implementation of dual polarization technology on the WSR-88D (Weather Surveillance Radar, 1988, Doppler) network, the US network of 159 radars used for weather observations. These data allow tornado-borne debris to be more readily detected even for short-lived, transient tornado vortices. In addition, the availability and quality of satellite imagery and uncrewed aerial system (UAS) platforms have dramatically improved the ability to detect tornadoes based on surface disturbances (e.g., swath marks, ground scouring, or debris deposition). Such satellite and UAS-based retrieval methods have been utilized in tandem with improved wind speed estimation techniques such as tree and crop fall analysis for near-surface wind field estimation following a tornado event. These techniques, along with direct observational techniques, allow comparisons with damage-based tornado wind speed estimations. The development of these techniques has allowed for increased confidence in estimations of the various relevant characteristics that vary spatially across the tornado vortex, and the plethora of datasets has enabled refinement and revamping of other methods based on these research findings. Finally, publicly available archival tools such as the National Oceanic and Atmospheric Administration (NOAA) Damage Assessment Toolkit (Camp et al. 2017) have increased the availability of data collected by the National Weather Service allowing for information on tornado damage dating nearly 15 years back to be easily acquired for analysis.
2.1.2 Tornado-Structure Interaction
The current state of the art in designing structures to resist tornado loads largely follows the methodology used to design structures to resist boundary layer winds. Differences in the methodology specific to tornado design include a higher value of the internal pressure to account for the effects of the APC within a tornado and a factor to account for the vertical winds within the tornado core. The effect of vertical winds, which is only applied to roof pressures, is accounted for using wind tunnel data from a boundary layer wind tunnel where the model building is tilted to artificially model the effects of changing angle of attack. Ideally, data obtained from tornado simulators should be incorporated into the loading coefficients specified in ASCE/SEI 7-22. The current approach is simplistic and likely ignores other effects specific to tornado-induced loads such as the effect of curvature of the horizontal flow, which could impact both wall and roof wind-induced pressures (as opposed to APC pressures).
In addition to the simplicity of the current approach, some aspects of the tornado-specific design guidance in ASCE/SEI 7-22 are inconsistent with published literature, are borrowed from research performed for hurricane-induced loading or are nonexistent. The pressure coefficients in the literature derived from tornado experiments are typically defined using the maximum tangential wind speed within the tornado (however, other definitions are also given in the literature), rather than the maximum horizontal wind speed, which is the wind speed specified in the ASCE/SEI 7-22 tornado load chapter. The difference between the total horizontal wind speed and the tangential wind speed in tornado simulators is likely small, owing to limitations of experimental facilities. However, real-world tornadoes can travel much faster than simulated tornadoes, increasing the difference between the tangential and total horizontal wind speed. Furthermore, real-world tornadoes show much stronger radial inflow near the ground. The tornado-borne debris requirements specified for use in ASCE/SEI 7-22 are based on hurricane design criteria and should be modified to represent the debris hazard expected in tornadoes. Similarly, currently no tornado-specific guidance is available on how to handle loads on flexible structures whose response has significant contributions due to resonance.
Generally, following the methodology to design structures to resist boundary layer winds also leads to some complications given current tornado simulator measuring capabilities. The experimentalists in the breakout group pointed out that when measuring pressures on the exterior of a building in a tornado simulator, accurately removing the contributions of the APC within the tornado from the wind-induced portion of the pressures is extremely difficult. Furthermore, characterizing the structure of the three-dimensional tornado wind field within a tornado simulator is extremely difficult, leading to complications in computing (GCp)’s defined in the traditional manner based on a 3-s gust wind speed where the definition of the gust wind speed in a tornado is itself unclear.
2.1.3 Design of Residential Structures
The current state of the art in tornado resistance for residential structures can be described as a tale of two realities because currently no requirements for residential structures to be designed for tornadoes exist. Some structures have many of the recommended features for enhanced performance in high wind events, and some structures are built using traditional techniques and rules of thumb. Many residential structures belonging to the former group are found in areas where modern codes have been adopted and are enforced. These regions are along the coasts where communities are also subjected to hurricane wind loads, thus the design parameters implemented for hurricane resistance also lend themselves to tornado resistance. In this discussion of tornadoes and residential buildings, worth noting is that hurricane-resistant design practices are used as a case study for tornado-resistant design due to greater data availability and maturity of hurricane-resistant design. Although hurricanes and tornadoes are distinct hazards, many of the lessons learned in increasing hurricane resistance can inform the development of tornado-resistant design for residential structures, especially for EF0–EF2 events, as defined by the Enhanced Fujita (EF) Scale (WiSE 2006).
Some states with effective state-wide building codes include Florida, Virginia, South Carolina, and California, among others. In many areas where state or local jurisdictional codes are lacking, residential construction that possesses features for better tornado performance are sporadic because individual builders and/or inspectors can choose whether to implement these improved structural measures. The social impacts of weaker construction techniques are made even worse when considering that homeowners do not know or understand if their home was designed, or enhanced, for tornado performance. These homeowners are often unaware that homes in many areas of the country are not designed or inspected for these components and attributes that would improve structural performance in any wind event including tornadoes.
Another frequently discussed topic in residential design is the resistance capacity of nonstructural elements and subassemblies such as roofing materials and garage doors that cannot withstand tornadoes with even relatively low wind speeds (such as an EF0) causing more dramatic damage to the structure with water infiltration and rapid internal pressure changes, respectively. Chimneys are also included in this list of commonly problematic subassemblies. Chimneys built in the 1950s and 1960s are quite brittle and can withstand little wind loading, and when they collapse, they can cause considerable damage to the both the roofing material and the roof sheathing depending on the extent of chimney collapse. Increasing the tornado resistance of these various elements could reduce secondary damage and degradation of a house.
In considering roofing further, the 2022 Hurricane Ian resulted in a large enough dataset of damaged roofs to provide statistically significant trends in roof type and age. These data show that age and durability have an inverse relationship for asphalt shingle roofs with damage becoming “almost certain” for roofs that are 15 years or older, yet this is not the case for other roofing materials (Giammanco et al. 2022). Furthermore, asphalt shingle failure is independent of nailing pattern and class but is a result of the asphalt's sealant failing. Although this poor wind resistance is known, insurance will only pay to replace with “like kind and quality,” leading homeowners who want to build back better with a more wind-resistant roofing material to have little to no option but to reinstall the type of roofing that resulted in damage during the event.
On the point of garage doors, the flexibility of widely available garage doors can allow them to temporarily deflect inward due to lateral loading and then rebound after the lateral loading subsides. This results in a breach of the building envelope during the event, leading to dramatic changes in internal pressure without even having a garage door failure. The clear solution to this is to install wind-rated garage doors; however, these are only available by special order at many stores around the country. Thus, this feeds into the continued theme from the roofing conversation in which more viable options are not available or economically accessible to homeowners.
One positive development in residential building design and construction is that adoption of select cost-efficient and commonly referenced damage prevention measures, such as the installation of hurricane straps in tornado-prone regions, is starting to increase. However, piecemeal adoption of these measures without ensuring the development of a continuous load path from the roof structure to the foundation can short-circuit the traditional failure sequence. In some cases, this can cause low wind events to result in catastrophic failures that have greater risk of jeopardizing life safety. For instance, this can happen when only the roof is strengthened, shifting failure down the structure such that the wall fails instead. As the effort to augment residential resistance to tornadic loading continues, it is interesting to consider how examples like this can inform not only structural research into residential design but also social science research into what leads to widespread adoption of certain recommended best practices and not others.
2.1.4 Design Using ASCE/SEI 7-22
ASCE/SEI 7-22 is the current state of the art for tornado provisions for practicing structural engineers. Specifically, the addition of Chapter 32 to ASCE/SEI 7-22 provides the most prescriptive methodology to obtain design loads for the most frequently observed tornadoes (EF0–EF2). Of roughly 1,200 tornadoes observed each year, approximately 97% are EF0–EF2. The addition of this new chapter in ASCE/SEI 7-22 was the culmination of years of observations and diligent documentation. The commentary of ASCE/SEI 7-22 summarizes the research that contributed to these provisions, which was based on tornado climatology and risk assessment studies by Twisdale et al. (2023), who document the development of tornado risk maps for building design in the United States.
The precursor to ASCE/SEI 7 was the American National Standards Institute publication ANSI A58.1 Minimum Design Loads for Buildings and Other Structures, first published in 1945. This document contained one paragraph and references on design for tornadoes in its commentary. This publication transitioned into ASCE/SEI 7, first released as ASCE/SEI 7-88. The tornado design guidance was expanded in ASCE/SEI 7-95 into two paragraphs within the wind chapter commentary, which described advancements in understanding the effects of tornadoes—labeled extraordinary events—on buildings. This understanding was, and still is today, achieved through collecting and analyzing data and cataloging damage to structures after tornadic storms. The observation was that in roughly one half of recorded tornadoes, gust speeds are less than the gust speeds associated with straight-line winds. The commentary points to several references to develop the loads and calls out a tornadic wind speed map first developed by the American Nuclear Society in the early 1980s (ANS 2016).
When ASCE/SEI 7 moved from service to ultimate wind speed maps in ASCE/SEI 7-10 (Section 26.5, Figures 26.5-1A through 26.5-1C), the wind chapters went through a reorganization, dividing wind load parameters into general provisions, the directional method, the envelope method, other structures and appurtenances, components and cladding, and wind tunnel testing. In the reorganization, a limitations section was added to the general provisions, stating, “Tornadoes have not been considered in developing the basic wind-speed distributions” (ASCE/SEI 7-10, Section 26.5.4). The commentary from previous editions was still included in the document, with further resources cited for tornado load evaluation.
Until the publication of ASCE/SEI 7-16, the standard was largely silent on how to calculate tornado loads, and ASCE/SEI 7-16 continued to have the same tornado limitation, simply stating that they were not considered in the wind load provisions. However, the commentary of ASCE/SEI 7-16 offered more than eight pages of guidance and methodology should a structural engineer want to incorporate tornado loads into a building design. Discussion included wind pressures induced by tornadoes versus other windstorms, tornado wind speeds and probabilities, designing for occupant protections, designing to minimize building damage, and designing to maintain continuity of building operations. Design examples were incorporated into this commentary.
The ASCE/SEI 7-16 commentary formed the basis of the new Chapter 32 in ASCE/SEI 7-22. Another key component was the assessments performed following the 2011 tornado in Joplin, Missouri (NIST 2014). Chapter 32, often called the tornado chapter, mirrors Chapter 26, the general provisions chapter, for ease of navigation by practitioners. Tornado maps are found within the same numbered section as the wind maps (32.5 and 26.5, respectively), for example. The beginning of the chapter contains a flow chart to guide users of the standard through when tornado load considerations are required in design. Only Risk Category III and IV structures must be evaluated for tornado design. The building or other structure must also be located within the tornado-prone region, which roughly includes all the United States east of the continental divide. If that condition is satisfied, then the tornado wind speed [obtained either through the maps in Section 32.5 or through the online ASCE Hazard Tool (ASCE n.d.)] must be greater than or equal to 60 mph (27 m/s). The tornado wind speed depends on the effective plan area of the structure because it correlates with the probability of a tornado strike. The larger the building, the higher the probability of a strike. The effective plan area differs from a building plan area because the effective plan area must encompass any ancillary structures that support the primary structure. Figure 2-1 shows an example from ASCE/SEI 7-22, which depicts a hospital and its accompanying central utility plant.
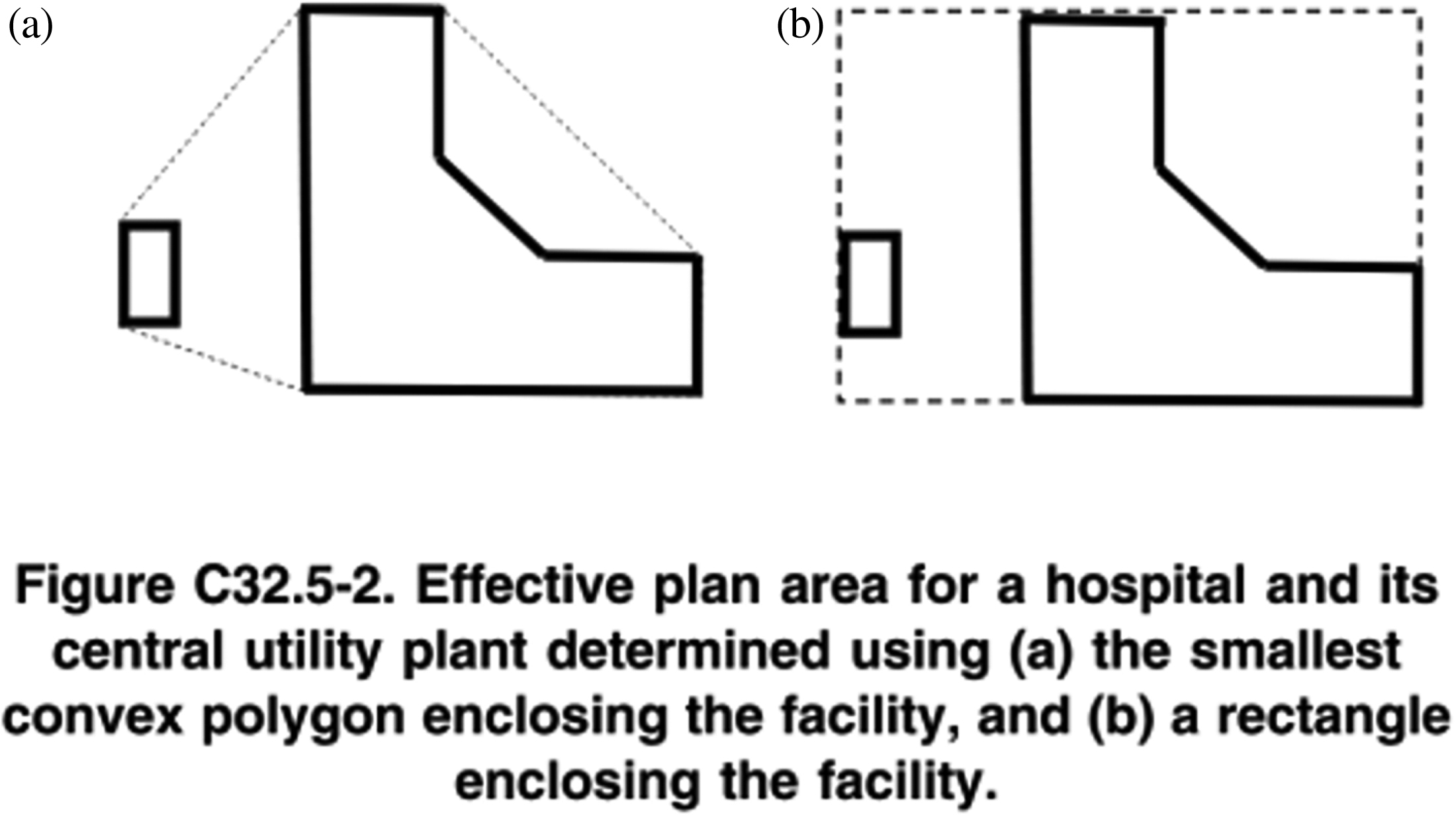
Figure 2-1. Example of effective plan area for tornado design.
Source: ASCE/SEI 7-22.
Assuming that the tornado wind speed is greater than 60 mph (27 m/s), then the last step is to compare the tornado wind speed from the maps in Chapter 32 with the mapped basic wind speed for straight-line winds from Chapter 26 in the same location. The building's or structure's exposure will dictate what percentage of wind speed is considered. The comparison is in place because observations found tornado loads will govern over wind loads at a given percentage of the basic wind speed for a given exposure. For example, exposure B tornado wind speed must be greater than or equal to half of the basic wind speed to require use of the tornado load design provisions. The process for including tornado loads in design is fairly similar to designing for straight-line winds, with one of the largest differences being in the internal pressure coefficient. For enclosed buildings, the straight-line wind internal positive pressure coefficient is +0.18. Because of the atmospheric pressure change associated with tornadoes, the same building must use a positive pressure coefficient of +0.55, when calculating tornado loads.
Several special provisions were included for Essential Facilities, which Chapter 1 of ASCE/SEI 7-22 defines as “Buildings and other structures that are intended to remain operational in the event of extreme environmental loading from flood, wind, tornado, snow, or earthquakes.” The tornado directionality factor KdT for components and cladding was set to 1.0 to reduce chances for damage to the building envelope that would permit wind, debris, and rain into the building. Other provisions intended to support the performance goal of maintaining operability following a design-level tornado event include requirements for essential facilities to protect glazed openings (e.g., impact-resistant glazing) and to consider multiple buildings/structures at the same facility in determination of the effective plan area, if all are required to maintain functionality.
NIST documented the impacts of the ASCE/SEI 7-22 tornado load requirements (Kneifel et al. 2022). This report includes the estimated percentage of buildings that would be affected and increases in the main wind force resisting system (MWFRS) and components and cladding loads over existing wind loads for four archetype buildings. Although tornado loads on some parts of the building can double or be more comparable to straight-line wind loads, cost increases were determined to be very modest, generally less than 0.15% of total construction costs. Typical impacts on design included modest increases in member size, additional anchorage, more fasteners for roof cladding, and similar. A design guide for the tornado load provisions of ASCE/SEI 7-22, jointly authored by FEMA and NIST (2023), summarizes the tornado load methodology, example calculations, and comparison with wind load calculations and design pressures.
Designing for tornadoes has made great strides within the past decade but additional work is needed. Topics such as effects of topography and exposure on tornado velocity pressure profiles are not as well understood when designing structures for tornado loads, though these two parameters are known to impact the structure of the tornado.
2.1.5 Design of Tornado Shelters and Safe Rooms
Current design practices for tornado shelters and safe rooms have succeeded in their fundamental goals of providing life safety protection from the most extreme tornadoes and other windstorms. Hundreds of storm shelters and safe rooms have been struck by tornadoes in the past two decades since the development of modern guidance for their design. “There has not been a single reported failure of a safe room constructed to FEMA criteria” (FEMA 2021a), nor has there been a documented failure of any storm shelter constructed to ICC 500 criteria.
Design requirements for tornado shelters are governed by the International Code Council's ICC/NSSA 500 Standard for the Design and Construction of Storm Shelters (ICC 2023). Loads used for tornado shelter design greatly exceed ASCE/SEI 7-22 Risk Category IV levels, given the intent of providing enhanced life safety for larger tornadoes (EF3–EF5). The load-related criteria in the latest edition of the storm shelter standard ICC 500 are based on ASCE/SEI 7-22, including the new Chapter 32 Tornado Load procedures, but with significant modifications. ICC 500 uses a deterministic tornado hazard map representing the near-worst-case scenario for maximum tornadic wind speeds in different regions of the country. This contrasts with ASCE/SEI 7-22, which uses multiple probabilistic tornado hazard maps depending on return period (tied to Risk Category) and effective plan area of the building or facility being designed. Other key ICC 500 modifications include use of a directionality factor of 1.0 in all cases and different treatment of atmospheric pressure change. All shelter surfaces must be evaluated to resist penetration from tornado-generated missiles (not just glazed openings), using faster and heavier missiles than required in ASCE/SEI 7-22 for hurricane windborne debris regions. Tornado shelters require a very robust 100 psf (4.78 kN/m2) roof live load (unreducible) and consideration of impact loads due to adjacent structure laydown and falling debris hazards. ICC 500 addresses many other aspects of storm shelter design beyond just loads, including architectural, mechanical, electrical, and plumbing considerations; special inspection; peer reviews; and to a limited extent, shelter operations and maintenance.
FEMA publishes guidance for the design of tornado safe rooms, which meet or exceed all storm shelter criteria (therefore, all safe rooms are also storm shelters). Following each new edition of the ICC 500 storm shelter standard, FEMA makes corresponding updates to its guidance for community safe rooms in FEMA P-361 Safe Rooms for Tornadoes and Hurricanes (FEMA 2021a) and for residential safe rooms in FEMA P-320 Taking Shelter from the Storm (FEMA 2021b). Updates to these guidance documents for the 2023 edition of the ICC 500 storm shelter standard are currently in development. FEMA P-361 includes extensive background and expanded commentary on ICC 500 provisions along with best practices. FEMA P-361 includes several additional technical requirements that are over and above ICC 500, referred to as FEMA funding criteria, which must be met to be eligible for FEMA funding to support safe room construction.
Following publication of the first edition of ICC 500 in 2008, the 2009 model building codes [the International Building Code (IBC) and the IRC] introduced requirements that storm shelters must meet the ICC 500 standard. However, the model codes did not require installation of tornado shelters. This changed with the publication of the 2015 IBC (ICC 2015), which began requiring storm shelters in schools and certain emergency response facilities located in the 250 mph (112 m/s) tornado shelter design wind speed zone. This zone encompasses the most tornado-prone areas of the country, covering all or parts of 22 states from the Great Plains to Texas to the Southeast and Midwest. Similar requirements for new buildings on existing school campuses and additions to existing school buildings were added in the 2018 IBC (ICC 2018a) and the International Existing Building Code (ICC 2018b), respectively.
2.1.6 Tornado Effects on Non-building Structures and Beyond ASCE/SEI 7-22
The state of the art of tornado design for non-building type structures varies by industry and organization. The workshop session focused on the industrial and liquified natural gas (LNG), nuclear and power, electric transmission and distribution, telecommunications, and solar industries, and on other non-building structures currently included in Section 32.16 of ASCE/SEI 7-22. Notably, many of the structures discussed in this session are not necessarily mandated to use ASCE/SEI 7-22 (i.e., not considered in the scope of ASCE/SEI 7-22), but rather the various industries have developed structure- and application-specific design procedures, guidelines, and standards. That said, many of these industries are exploring the application and implications of the tornado load framework in Chapter 32 of ASCE/SEI 7-22. For many of these structures, failure is unlikely to directly endanger lives; however, failure could result in subsequent impact to communities in the immediate vicinity of these structures.
The state of the art for LNG facilities revolves around the implementation of 88 FR 74033 by the Federal Energy Regulatory Commission (FERC), December 2023. This document requires facilities to provide studies and/or basis of design for tornadic events for LNG facilities. The design basis for the tornado wind speeds and windborne missile requirements for the impounding system of storage tanks come from various codes and standards, including federal and state regulations and requirements. Title 49 CFR § 193.2067 requires LNG facilities to be designed to withstand, without loss of structural or functional integrity, the direct effect of wind forces based on an assumed sustained wind velocity of not less than 150 mph (67 m/s) or the most critical combination of wind velocity and duration having a probability of exceedance in a 50-year period of 0.5% or less. Title 49 CFR. § 193.2067(b)(1) incorporates by reference ASCE/SEI 7-05 (ASCE 2005) for applicable wind load data for shop-fabricated containers of LNG or other hazardous fluids with a capacity of not more than 70,000 gal. (265 m3). The balance of the LNG facility is typically designed around a 10,000-year MRI referencing ASCE/SEI 7-22 for the straight-line wind loading development process. LNG facilities also follow ASCE/SEI 7-22 design criteria to determine tornado loads.
Similarly, nuclear and power facilities exceed the requirements of the tornado provisions in ASCE/SEI 7-22 under guidance from the US Nuclear Regulatory Commission (NRC). NUREG-0800 SRP (NRC 2007b) is the standard review plan (SRP) that provides regulatory review guidelines on tornado loading requirements. The latest version 2 of the SRP, issued in 2007, points to NRC Regulatory Guide 1.76 Rev. 1 (RG 1.76; NRC 2007a) and ASCE/SEI 7-05. RG 1.76 outlines design methods “acceptable for use in selecting the design-basis tornado and design-basis tornado-generated missiles that a nuclear power plant should be designed to withstand to prevent undue risk to the health and safety of the public.” Based on geographical region, RG 1.76 specifies deterministic design tornado wind speeds and applicable tornado-borne missile criteria such as dimensions, mass, and horizontal speed to be incorporated into the design. The design-basis tornado wind speeds presented in the regulatory guide are based on NUREG/CR-4461 revision 2 (NRC 2007c). The tornado database used in NUREG/CR-4461 includes information recorded for more than 46,800 tornado segments occurring from January 1, 1950, through August 31, 2003. However, RG 1.76 and the SRP are more than a decade old and still reference ASCE/SEI 7-05 for straight-line wind load development.
The design of electric transmission and distribution networks typically follows a combination of ASCE Manual of Practice 74 (ASCE 2020) and the National Electric Safety Code (NESC; IEEE SA 2023) for transmission and distribution structures and ASCE Manual of Practice 113 (ASCE 2023a) for substations because these structures are excluded in the scope of ASCE/SEI 7-22. It is important to note that distribution structures under 60 ft tall are exempt from extreme wind loading acting on the conductors per NESC Rule 250C. In the current version of ASCE Manual of Practice 74, design procedures for tornado winds are included in Appendix K; however, these additional loads are not mandatory and generally are not incorporated unless dictated by client preference. Appendix K includes simplified and detailed approaches for considering tornado load cases on transmission structures. The load cases are benchmarked to a high-end F2 (Fujita Scale) tornado in terms of wind speed [i.e., 161 mph (72 m/s)] and account for the simultaneous effects of wind from various directions characterized by tornado wind fields. ASCE Manual of Practice 113 does not currently provide guidance on tornado design provisions and for straight-line wind design and specifies a 300-year MRI wind speed for ultimate strength design and a 100-year MRI wind speed for allowable stress design of substation structures. Both ASCE Manual of Practice 74 and the 2023 NESC use a 100-year MRI as the nominal basis of design. Notably, the wind loads are developed differently in each of these documents compared with the provisions in ASCE/SEI 7-22, which complicates a comparison of wind loading criteria based solely on wind map MRI. An ASCE Standard on Minimum Loads for Overhead Power Lines is currently under preparation.
Telecommunications structures follow the Telecommunications Industry Association's (TIA's) Structural Standard for Antenna Supporting Structures, Antennas, and Small Wind Turbine Support Structures (ANSI/TIA-222, TIA 2019) as referenced in the IBC and are remarkably similar in their current state of the art for tornado design as transmission structures. ANSI/TIA-222 provides conventional straight-line wind, ice, seismic, and operational load requirements, consistent with ASCE/SEI 7-22 and standards from the American Concrete Institute and the American Institute of Steel Construction. Telecommunications towers historically have lacked tornado provisions within their design guidelines. Tornado loads were not previously considered in the design process as a tornadic event is considered an extraordinary event. However, the latest revision of ANSI/TIA-222, Revision I, does adopt recommendations from ASCE/SEI 7-22 for tornado loads.
Lastly, design of solar photovoltaic (PV) facilities could be characterized as the “Wild West” due to a lack of explicit code design guidance for solar industry structures. For this reason, the state of the art for solar structures direly needs improvement, especially regarding tornado design provisions. An ASCE/SEI Manual of Practice is currently being developed for structural design guidance in the solar PV field; however, tornado loading is not anticipated to be included. The solar tornado provisions in ASCE/SEI 7-22 were developed based on the tornado wind field characteristics as they relate to buildings. However, more guidance is needed that is specific to roof-mounted and ground-mounted solar arrays due to a lack of research.
2.2 Long-Term Vision for Tornado Design
Each breakout group was asked to give its estimate of the status of tornado design 10 years from now.
2.2.1 Tornado Climatology and Near-Surface Wind Characteristics
Considering the progress of the previous decade, the long-term vision of tornado-based structural design focuses on improving the understanding of the climatology of tornadoes such that the risk related to tornadoes can be properly quantified for implementation in design standards and the near-surface wind characteristics to understand the loads directly imparted by tornadoes on structures.
The current deficiencies in the understanding of the near-surface flow field in tornadoes generally stem from a lack of direct measurements. Though the number of datasets has rapidly increased in previous years, additional observation of tornadoes from multiple instruments (e.g., in situ, radar, etc.) is needed to expand the datasets available for making informed decisions regarding all engineering-relevant parameters. Ideally, these observations would be of high resolution in both space and time to capture the turbulent properties of tornado flow. The focus for observations should consider the spectrum of scales of motion, both spatially and temporally from tornado scale to storm scale. Currently, turbulence data for tornado flows remain largely limited to laboratory and numerical simulations with little-to-no formal verification in real tornadoes.
Specifically, additional emphasis should be placed on capturing a range/envelope of loading-relevant parameters. These quantities include flow curvature and acceleration, pressure gradients, translation, vortex structure (e.g., swirl ratio), vertical wind components, and the vertical profile of the horizontal winds within tornado vortices. Most understanding of these parameters remains tied to estimations from simulations (laboratory and numerical) or from remote sensing techniques involving major assumptions (e.g., mass continuity assumptions for dual-Doppler radar analysis). Direct observations are highly desired; however, these are difficult to accomplish for many reasons, mostly logistical. Such goals could be accomplished through additional observations of tornadoes to add to those collected in the past, thereby increasing the total number of observations, and integration of observational data with high-resolution numerical simulations via data assimilation techniques. In addition, novel research avenues such as dust devil observations (e.g., Bluestein et al. 2004, Lombardo et al. 2023) could be used to help bridge the gap between laboratory simulations and reality. Further, the interaction of tornadoes with surface obstacles such as structures, terrain, and topography are necessary to understand the potential impacts of tornado-obstacle feedback (both with respect to the impacts on the structure and ultimately the dynamic response of the tornado vortex) and how drastic variances in surface roughness can alter the near-surface flow.
From a climatological perspective, most tornado data collection currently occurs post-event. However, these data collection efforts, whether through the research community or National Weather Service partners, often lack consistency in terms of the data types, quality, engineering observations (i.e., determination of load path failure from detailed observation), and the degree of detail observed from these events. In the future, standardizing data collection efforts and developing tools and methods to store such data (e.g., the NOAA Damage Assessment Toolkit) for future access must be heavily emphasized. More rigorous, engineer-informed post-tornado data collection practices should be explicitly defined such that all tornado events can be more easily compared and the various methods for wind speed estimation can be applied and compared. In addition, with more rigorous data collection practices, these events can be reanalyzed in the future as innovative technologies and techniques continue to emerge (e.g., artificial intelligence and machine learning) and as we aim to further characterize future tornado risk in a changing climate.
2.2.2 Tornado-Structure Interaction
Long-term goals for tornado design with respect to tornado-structure interaction include the incorporation of data from tornado simulator test results, numerical modeling, or other innovative methods, consistent with the design philosophy and approach used in ASCE/SEI 7-22. Furthermore, risk-consistent tornado missile impact and pressure requirements (including the atmospheric pressure drop and the tornado wind-induced pressures) need to be developed. The design criteria should be tornado wind speed dependent to capture the variation in the tornado-borne debris hazard as a function of tornado wind speed. The current windborne debris requirements, for both hurricanes and tornadoes, do not accurately reflect the change in the windborne debris requirements with changes in the design wind speeds.
Both physical testing and numerical modeling will play a role in meeting these long-term goals. To produce reliable experimental results, a standard is needed for tornado simulators, similar to, or within, the ASCE/SEI 49-21 (ASCE 2021) standard for wind tunnel testing, to ensure that minimum requirements in terms of scaling, flow characteristics, instrumentation, repeatability, and so on, are met. ASCE/SEI 49 does cover tornadoes but as of the 2021 edition indicated that consensus did not yet exist on the requirements for tornado simulators. Additionally, a standard model building to be tested in each tornado simulator facility, similar to the CAARC (Commonwealth Advisory Aeronautical Research Council) high-rise or Aylesbury low-rise buildings, tested in multiple boundary layer wind tunnels is desirable. Numerical modeling may provide a means to eliminate many challenges of repeatability issues associated with experiments performed in tornado simulators. Numerical modeling may also allow for the separation of the effects of the negative atmospheric pressure (e.g., APC) in the tornado and the velocity-induced portion [i.e., (GCp)] of the tornado loads and could shed light on the effect of the APC gradient on tornado-induced pressure due to the relative size and position of a structure to the tornado.
2.2.3 Design of Residential Structures
The vision for tornado resilience for residential structures includes design and construction of individual homes and neighborhoods to be able to survive lower-level (EF0–EF2) tornadoes and debris damage without catastrophic loss to homeowners and communities. A primary goal when considering future residential design is community resilience and the ability to disaggregate community-level objectives to individual housing unit objectives. Community resilience for tornadoes largely stems from the ability of the local housing stock to withstand and recover from tornadic wind loading because these structures constitute such a large percentage of the building stock in a community and are needed to house individuals following an event. Although residential structures cannot be expected to withstand windspeeds and resulting pressures generally associated with an EF5 tornado, more can be done to limit damage due to EF0–EF2 tornadoes. Furthermore, studies show that even in high wind speed tornadoes the area of the community subjected to peak windspeeds remains concentrated within the tornado's radius of maximum wind, while much of the surrounding area experiences EF0–EF2 windspeeds (van de Lindt et al. 2013). If a dual objective and/or performance-based design approach is adopted, in which limiting damage is the design goal for lower-end windspeeds and preserving life safety is the design goal for high-end windspeeds, the result would be communities with much fewer uninhabitable housing units after an EF5 and virtually no uninhabitable housing units after an EF2. Just by reducing the number of drastically impacted households in this way, the built environment could augment community resilience. Ultimately, design of residential structures, including wood frame, will need to consider tornado loading as a requirement in building codes. This may be a loading at EF2 wind speeds, or another level may be considered, but that is part of the research questions that need to be answered. This work could be informed by the work from the Canadian Standards Association Group with their standard CSA S520:22, Design and Construction of Low-Rise Residential and Small Buildings to Resist High Wind (CSA Group 2022).
Another key advancement would be a shift away from individual element failure and toward a system-level approach. Some shift toward this has occurred in recent years, but this should become the primary way in which residential structures are tested, designed, and constructed. This will allow for the ability to model how load transfers through diverse residential buildings of all construction types. It would also enable the exploration of varying reliability by component or subassembly to allow for and predict failure sequences that minimize damage, preserve life safety, and/or maintain top-down failures. A conversation about system-level analyses would be incomplete if it considered only new construction in these analyses. Also essential is that this vision for the future includes a guide (or standard ideally) to retrofit existing residential structures for tornadoes. This type of guide or standard should include comprehensive retrofitting that may be invasive but should also consider approaches that are less disruptive to owners and tenants that may not achieve as good a performance as the fully comprehensive approach. Thus, developing a retrofitting guide or standard is imperative to protect the entire community, not just those living in houses constructed after a given code adoption date.
Closely connected to the conversation of building retrofit is the need to create a shift in the enfranchisement of homeowners to not only know what they are buying but also what options are available to them to increase the tornado resistance of homes they already own. This can be improved in a few ways.
First and foremost, more efficient and accurate home inspection processes would aid in providing this information to homebuyers. The home inspector community is stretched too thin in many areas, and thus homeowners and inspectors may benefit from implementing artificial intelligence and remote sensing to assess homes using advanced technology that will allow the inspector to drastically reduce their time on site while opening the possibility that at some point in the future, inspections could be done fully remotely.
Second, a home appraisal should consider the fortification measures in place to reward homeowners who have taken proactive steps to protect against an event that may or may not occur while they own the house. These measures are a value added for the next buyer and should be considered as such. Lastly, for situations where tornado retrofits or improved new construction may be difficult for the free market to bear, diverse incentive pathways should be in place in the form of insurance cost adjustments, property tax reductions, or other means. These steps will allow the gap between research-backed best practice and what is happening in communities to be bridged.
2.2.4 Design Using ASCE/SEI 7-22
To the users of ASCE/SEI 7-22—practicing structural engineers—the long-term vision of tornado design is all-encompassing and folds in future research meant to better define tornado loads. The current provisions only include reviewing Risk Category II structures, such as office buildings, or warehouses that may not have a large occupancy but store critical objects, such as medicine. This also relates to the category of tornado considered. The current standard is based on tornado wind speeds associated with EF0–EF2 tornadoes. While those are the most frequently occurring tornadoes, the EF3–EF5 tornadoes individually cause much more damage. Incorporating research discussed in other sections of this report, such as an improved understanding of windborne debris due to tornadoes and other effects like topography and exposure, would be included in the long-term vision for the standard.
In addition to the current standards, providing designers with guidance on how to address retrofitting existing structures for tornado loads would be useful in future guidelines or standards. Some clients, and most in the public, do not understand the relationship between straight-line wind speeds and tornado wind speeds, versus the EF categories with associated speeds used in news reports after a tornado strike. In the long term, additional guidance for designers and improved communication and education efforts for the public to connect the understanding of a building's or structure's resistance to a particular EF tornado would be helpful.
A long-term goal is consistency for tornado design across jurisdictions. Where building codes are adopted, the Authority Having Jurisdiction is permitted to adopt the provisions they feel are most appropriate. These vary from state to state but, more importantly, town to town, and regulations across city or county lines could mean the difference in design requirements. This ties back to educating the public, including building officials, about the latest design codes and standards and the importance of their adoption. Houston, Texas, is leading the way by adopting ASCE/SEI 7-22. Local structural engineers worked with city building officials so that they could understand the implications. The city realized the betterment and adopted ASCE/SEI 7-22. Federal design codes, namely the Unified Facilities Criteria (UFC, DOD 2024), are typically reviewed quarterly for the latest updates to building codes and are often among the first to adopt the latest ICC standards.
ASCE/SEI 7-22 is a load standard focused on new construction. However, the question of how to treat building retrofits, renovations, additions, and/or envelope replacement projects invariably arises. To what extent can tornado provisions be applied? Design guidance for existing building work will assist structural engineers in their assessment of a roof replacement project at its 25-year end of life, when the building itself still has another 25 years of service life.
Long-term, designers would have a better understanding of tornado-borne debris and its literal impact on buildings and structures. The question of requirements to design for debris impacts, which include glazing and other elements of a building envelope, would be solved.
In 2019, ASCE/SEI published Prestandard for Performance-Based Design for Wind, with V1.1 following in 2023 (ASCE 2023b), and performance-based design for tornadoes should also be considered in long-term tornado design. Similarly, further developing tornado simulators will help to identify the differences between straight-line winds and tornadoes.
2.2.5 Design of Tornado Shelters and Safe Rooms
The vision for tornado shelter and safe room design centers around balancing “engineering successes” with economical solutions in the design of these structures. Because shelters and safe rooms are more expensive than typical construction, providing lower-cost and easily implementable options and structuring public awareness campaigns around these practical approaches will increase the construction of these structures and ultimately reduce fatalities in tornadoes. To reach these goals, much research is needed.
One emerging area that would benefit future designers is guidance for larger storm shelters and safe rooms. Architects are constantly pushing for larger protective structures, and the tornado wind loading on these larger structures often leads to increased structural member sizes and smaller usable space within the structures. The long-term vision for the design of these shelters includes better understanding of the wind loading on structural members in larger protective structures and more off-the-shelf tested and understood assemblies that designers can use in their designs.
A point of emphasis moving forward is the coordination among industries and clarification of provisions when multiple disciplinary standards are used in design. In particular, standards would address the handshake among architects, structural designers, and mechanical/electrical/plumbing engineers to ensure that requirements of nonstructural standards do not cause conflicts with the structural design of projects.
Consideration of human needs in storm shelters and safe rooms is critical. Improved fire and smoke guidance or requirements within storm shelters and safe rooms for post-event duration life safety are needed to ensure the safe operation of these facilities. For example, if a host structure fails in a tornado causing a fire adjacent to a protective structure, occupants may not have a safe method of egress. Dampers allow air flow into the structure, which would also allow smoke to enter. This idea can be summarized as “let me breathe or let me leave.” Similarly, the future vision for protective structures would include development of occupation, duration, space, and ventilation needs to ensure occupant safety in protective structures given the potential for extended duration in these structures.
The public is typically unaware of the differences between traditional structures and storm shelters and safe rooms, which could create situations where people seek shelter in areas that they think are safe but are not designed according to ICC 500 or FEMA guidelines. Therefore, a need exists to identify and create consistent signage for existing “shelters” that precede engineering design standards for protective structures. This would require a robust public awareness campaign to ensure that the public understands where they can expect to be safe in tornadoes.
Another future goal for tornado design of storm shelters and safe rooms is a move away from the deterministic wind speed maps that currently inform design and toward probabilistic maps to align storm shelter and safe room design with the new ASCE/SEI 7-22 tornado wind speed maps. Additionally, a better understanding of tornado wind field characteristics and how to account for their complexity is a goal in the next decade. As with all structures, a future goal for storm shelters and safe rooms is better adoption and enforcement of protective structure design triggers and criteria for tornado-prone regions in the United States. Without appropriate application of these criteria, tornado risk will continue to increase with future development.
2.2.6 Tornado Effects on Non-building Structures and Beyond ASCE/SEI 7-22
The vision for tornado design varies across industries. While LNG and other critical industrial facilities already have guidance from the FERC on tornado load development and requirements, a desire exists for ASCE/SEI 7-22 to adopt similar design approaches regarding requirements for site-specific tornado studies and implementation of standardized tornado-borne missile loading, potentially as a function of the Risk Category. These requirements could then be incorporated into the design of LNG facilities outside of the impounding system of LNG storage tanks.
Nuclear and power facilities are already governed by more robust design requirements for tornado loading than ASCE/SEI 7-22 provides for. The long-term vision for nuclear and power facilities is to align with the long-return period mapping developed in ASCE/SEI 7-22 and further develop the tornado provisions in ASCE/SEI 7-22 to increase the reliability and strengthen the requirements of tornado design to a level that meets NRC adoption. This would create a consolidated design code applicable to most, if not all, non-building structure requirements and eliminate the need for industry-specific tornado design guides. However, the long-term vision for tornado design within the transmission and distribution, telecommunications, and solar industries is different and perhaps behind the more critical infrastructure of LNG and nuclear and power facilities. Aspects under consideration in this regard also include resiliency planning, redundancy, and restoration ability. Without adopted design codes for these industries, the long-term vision for tornado design would first include incorporation of existing design manuals into local building codes. In the interim, these industries could begin implementing tornado provisions into design practice through ASCE/SEI 7-22, while continuing research and development of design approaches to ensure they are accurate and efficient for non-building structures. Ultimately, the long-term vision for all non-building structure industries hinges on the further development of ASCE/SEI 7-22 tornado provisions and whether specific load cases should be considered for specific structures to capture unique vulnerabilities.
For structures specific to ASCE/SEI 7, the goals are to include tornado missile debris requirements and to improve understanding of the scale and magnitude of atmospheric pressure change on tanks, specifically larger-diameter tanks. Missile debris requirements often govern design in the LNG and nuclear industries, and atmospheric pressure change can be amplified in tanks where the internal envelope is essentially sealed. Through future revisions of ASCE/SEI 7, a better understanding of the intention of the tornado provisions and how these provisions provide resilience for communities needs to be explicitly illustrated to assist in justifying any additional costs. By investing in the aforementioned discussion points, the structural engineering community can stand behind unified requirements in ASCE/SEI 7-22 that provides adequate information to serve all industries for tornado design.
References
ANS (American Nuclear Society). 2016. Estimating tornado, hurricane, and extreme straight-line wind characteristics at nuclear facility sites. ANSI/ANS 2.3-2011 (R2016). La Grange Park, IL: ANS.
ANSI (American National Standards Institute). 1945. Minimum design loads for buildings and other structures. ANSI A58.1. Washington, DC: ANSI.
ASCE. 1988. Minimum design loads for buildings and other structures. ASCE/SEI 7-88. Reston, VA: ASCE.
ASCE. 1995. Minimum design loads for buildings and other structures. ASCE/SEI 7-95. Reston, VA: ASCE.
ASCE. 2005. Minimum design loads for buildings and other structures. ASCE/SEI 7-05. Reston, VA: ASCE.
ASCE. 2010. Minimum design loads for buildings and other structures. ASCE/SEI 7-10. Reston, VA: ASCE.
ASCE. 2016. Minimum design loads and associated criteria for buildings and other structures. ASCE/SEI 7-16. Reston, VA: ASCE.
ASCE. 2019. Prestandard for performance-based design for wind, V1.0. Reston, VA: ASCE.
ASCE. 2020. Guidelines for electrical transmission line structural loading, 4th ed. Manual of Practice 74. Reston, VA: ASCE.
ASCE. 2021. Wind tunnel testing for buildings and other structures. ASCE/SEI 49-21. Reston, VA: ASCE.
ASCE. 2022. Minimum design loads and associated criteria for buildings and other structures. ASCE/SEI 7-22. Reston, VA: ASCE.
ASCE. 2023a. Substation structure design guide, 2nd ed. Manual of Practice 113. Reston, VA: ASCE.
ASCE. 2023b. Prestandard for performance-based design for wind, V1.1. Reston, VA: ASCE.
ASCE. n.d. Hazard tool. Reston, VA: ASCE.
Bluestein, H. B., C. C. Weiss, and A. L. Pazmany. 2004. “Doppler radar observations of dust devils in Texas.” Mon. Wea. Rev. 132 (1): 209–224.
Bodine, D. J., and C. B. Griffin. 2023. “Meteorological research enabled by rapid-scan radar technology.” Mon. Wea. Rev. 152 (1): 3–37.
Bodine, D. J., T. Maruyama, R. D. Palmer, C. J. Fulton, et al. 2016. “Sensitivity of tornado dynamics to soil debris loading.” J. Atmos. Sci. 73 (7): 2783–2801.
Bryan, G. H., N. A. Dahl, D. S. Nolan, and R. Rotunno. 2017. “An eddy injection method for large-eddy simulations of tornado-like vortices.” Mon. Wea. Rev. 145 (5): 1937–1961.
Camp, J. P., P. Kirkwood, J. G. LaDue, L. A. Schultz, et al. 2017. “National Weather Service damage assessment toolkit: Transitioning to operations.” Abstract 9.1. In Proc., Annual Meeting. Boston: American Meteorological Society.
Code of Federal Regulations. n.d. “Title 49 of the Code of Federal Regulations.” https://www.ecfr.gov/current/title-49.
CSA Group (Canadian Standards Association Group). 2022. Design and construction of low-rise residential and small buildings to resist high wind. CSA S520:22. Mississauga, ON: CSA Group.
DOD (US Department of Defense). 2024. “Department of Defense standard (UFC) and Unified Facilities Guide specifications (UFGS).” Whole Building Design Guide. Accessed November 1, 2019. https://www.wbdg.org/ffc/dod/unified-facilities-guide-specifications-ufgs.
FEMA (Federal Emergency Management Agency). 2021a. Safe Rooms for Tornadoes and Hurricanes: Design and construction guidance for community safe rooms, 4th ed. FEMA P-361. Washington, DC: FEMA.
FEMA. 2021b. Taking shelter from the storm: Building or installing a safe room for your home, 5th ed. FEMA P-320. Washington, DC: FEMA.
FEMA and NIST (Federal Emergency Management Agency and National Institute of Standards and Technology). 2023. Design guide for new tornado load requirements in ASCE 7-22. Washington, DC: FEMA.
FERC (Federal Energy Regulatory Commission). 2023. Engineering and design materials for liquified natural gas facilities related to potential impacts caused by natural hazards. 88 FR 74033. Washington, DC: FERC, Department of Energy.
Giammanco, I. M., E. Newby, and W. H. Pogorzelski. 2022. Observations of building performance in Southwest Florida during Hurricane Ian (2022): Part I: Roof cover damage assessment on residential and light commercial structures. Technical Report. Richburg, SC: Insurance Institute for Business and Home Safety.
ICC (International Code Council). 2015. International building code. Country Club Hills, IL: ICC.
ICC. 2018a. International building code. Country Club Hills, IL: ICC.
ICC. 2018b. International existing building code. Country Club Hills, IL: ICC.
ICC. 2021. IRC: International residential code for one- and two-family dwellings. Country Club Hills, IL: ICC.
ICC. 2023. ICC/NSSA standard for the design and construction of storm shelters. ICC 500. Country Club Hills, IL: ICC.
IEEE SA (IEEE Standard Association). 2023. “National electric safety code.” ANSI standard C2. Accessed February 1, 2023. https://standards.ieee.org/products-programs/nesc/.
Kneifel, J., M. Levitan, B. Haney, B. Harris, et al. 2022. Economic analysis of ASCE 7-22 tornado load requirements. NIST TN 2214. Gaithersburg, MD: NIST.
Lombardo, F. T., A. Zaldivar de Alba, G. Chen, D. M. Rhee, et al. 2023. “An atmospheric vortex and its induced loading on a bluff body.” J. Wind Eng. Ind. Aerodyn. 243: 105605.
McKeown, K. E., M. M. French, K. S. Tuftedal, D. M. Kingfield, et al. 2020. “Rapid-scan and polarimetric radar observations of the dissipation of a violent tornado on 9 May 2016 near Sulphur, Oklahoma.” Mon. Wea. Rev. 148 (9): 3951–3971.
NIST (National Institute of Standards and Technology). 2014. Technical investigation of the May 22, 2011, tornado in Joplin, Missouri. Final Report. Gaithersburg, MD: NIST.
Nolan, D. S., N. A. Dahl, G. H. Bryan, and R. Rotunno. 2017. “Tornado vortex structure, intensity, and surface wind gusts in large-eddy simulations with fully developed turbulence.” J. Atmos. Sci. 74 (5): 1573–1597.
NRC (US Nuclear Regulatory Commission). 2007a. Design-basis tornado and tornado missiles for nuclear power plants. Regulatory guide 1.76. Washington, DC: NRC.
NRC. 2007b. Standard review plan for the review of safety analysis reports for nuclear power plants: LWR edition. NUREG-0800. Washington, DC: NRC.
NRC. 2007c. Tornado climatology of the contiguous United States, revision 2. NUREG/CR-4461, Revision 2. Washington, DC: NRC.
Satrio, M. A., D. J. Bodine, A. E. Reinhart, T. Maruyama, et al. 2020. “Understanding how complex terrain impacts tornado dynamics using a suite of high resolution numerical simulations.” J. Atmos. Sci. 77 (10): 3277–3300.
TIA (Telecommunications Industry Association). 2019. Structural standard for antenna supporting structures, antennas, and small wind turbine support structures. ANSI/TIA 222-H-1. Arlington, VA: TIA.
Twisdale, L., S. Banik, L. Mudd, M. Hardy, et al. 2023. Tornado wind speed maps for building design: Research and development of tornado risk assessment methodology. NIST TN 224. Gaithersburg, MD: NIST.
van de Lindt, J. W., S. Pei, T. Dao, A. Graettinger, et al. 2013. “Dual-objective-based tornado design Philosophy.” J. Structr. Eng. 139 (2): 251–263.
WiSE (Wind Science and Engineering). 2006. “A recommendation for an Enhanced Fujita Scale, Rev.2, Wind Science and Engineering Research Center, Oct. 2006.” Accessed May 1, 2024. http://www.wind.ttu.edu/EFScale.pdf.
Wurman, J., K. Kosiba, and P. Robinson. 2013. “In situ, Doppler radar, and video observations of the interior structure of a tornado and the wind–damage relationship.” Bull. Am. Meteorol. Soc. 94 (6): 835–846.
Information & Authors
Information
Published In
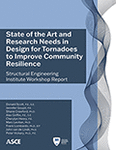
State of the Art and Research Needs in Design for Tornadoes to Improve Community Resilience: Structural Engineering Institute Workshop Report
Pages: 7 - 22
ISBN (Online): 978-0-7844-8582-8
Copyright
© 2024 by the American Society of Civil Engineers.
History
Published online: Sep 23, 2024
ASCE Technical Topics:
Authors
Metrics & Citations
Metrics
Citations
Download citation
If you have the appropriate software installed, you can download article citation data to the citation manager of your choice. Simply select your manager software from the list below and click Download.